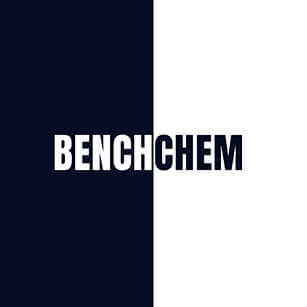
Cuprous iodide
- 点击 快速询问 获取最新报价。
- 提供有竞争力价格的高质量产品,您可以更专注于研究。
描述
Cuprous iodide, also known as copper(I) iodide, is an inorganic compound with the chemical formula CuI. It is a white solid that often appears tan or reddish-brown due to impurities. This compound is known for its diverse applications, ranging from organic synthesis to cloud seeding .
准备方法
Synthetic Routes and Reaction Conditions: Cuprous iodide can be prepared through several methods:
Direct Combination: Heating copper and iodine in concentrated hydroiodic acid.
Precipitation Method: Mixing an aqueous solution of potassium iodide with a soluble copper(II) salt, such as copper(II) sulfate.
Nanoparticle Synthesis: Reacting copper(II) sulfate with potassium iodide in ethanol solutions, yielding this compound nanoparticles.
Industrial Production Methods: In industrial settings, this compound is often produced by the direct combination method due to its simplicity and efficiency. The reaction is typically carried out under controlled conditions to ensure high purity and yield.
化学反应分析
Cuprous iodide undergoes various types of chemical reactions:
Oxidation: this compound can be oxidized to copper(II) iodide in the presence of oxidizing agents.
Reduction: It can be reduced back to elemental copper using reducing agents.
Substitution: this compound reacts with mercury vapors to form copper(I) tetraiodomercurate(II) ([Cu+]2[HgI4]2−).
Common Reagents and Conditions:
Oxidizing Agents: Oxygen, hydrogen peroxide.
Reducing Agents: Hydrogen gas, sodium borohydride.
Substitution Reactions: Mercury vapors.
Major Products:
Oxidation: Copper(II) iodide.
Reduction: Elemental copper.
Substitution: Copper(I) tetraiodomercurate(II).
科学研究应用
Catalysis in Organic Synthesis
Cuprous iodide is widely recognized for its role as a catalyst in organic synthesis. It facilitates several important reactions, particularly in the formation of carbon-carbon bonds through cross-coupling reactions. Notable applications include:
- Ullmann Coupling : this compound mediates C–N, C–O, and C–S bond formations, which are essential transformations in organic chemistry. This method offers a more economical and efficient approach compared to traditional methods that require harsher conditions .
- Polymerization Reactions : this compound is employed in radical polymerizations and the synthesis of copolymers. Its ability to control polymer architecture is crucial for developing new materials .
Antibacterial Properties
Recent studies have highlighted the antibacterial activity of this compound nanoparticles. These nanoparticles exhibit significant efficacy against both gram-positive and gram-negative bacteria by generating reactive oxygen species (ROS), which lead to bacterial cell death through oxidative stress mechanisms . Key findings include:
- Minimum Inhibitory Concentration (MIC) : The MIC values for various bacterial strains indicate that this compound nanoparticles are particularly effective against sensitive strains like Escherichia coli (DH5α), with MIC values as low as 0.066 mg/ml .
Semiconductor Applications
This compound is an intrinsic p-type semiconductor with promising optoelectronic properties. It has been investigated for use in:
- Solar Cells : this compound serves as a hole transport material in perovskite solar cells, achieving efficiencies exceeding 21% at room temperature . Its compatibility with silicon substrates enhances its potential for integration into existing solar technologies.
- Light Emitting Devices : The compound exhibits strong photoluminescence and has been explored for use in hybrid light-emitting devices due to its favorable electronic properties .
Medical Applications
In the medical field, this compound has been incorporated into dietary supplements as a source of iodine, crucial for thyroid function. The Food and Drug Administration recognizes its use in table salt to prevent iodine deficiency . Additionally:
- Radioactive Iodine Therapy : While not directly related to this compound, iodine isotopes play a significant role in thyroid cancer treatment, illustrating the broader relevance of iodine compounds in medicine .
Environmental Applications
This compound has been utilized in cloud seeding technologies aimed at enhancing precipitation. Its ability to act as a catalyst in atmospheric processes contributes to artificial rainfall efforts, showcasing its environmental significance .
Data Summary Table
Application Area | Specific Use Case | Key Findings/Properties |
---|---|---|
Organic Synthesis | Ullmann Coupling | Efficient C–N, C–O, C–S bond formation |
Polymerization | Control over polymer architecture | |
Antibacterial Activity | Nanoparticles | Effective against gram-positive/negative bacteria |
Semiconductor | Solar Cells | Hole transport material with >21% efficiency |
Light Emitting Devices | Strong photoluminescence | |
Medical | Dietary Supplements | Source of iodine for thyroid health |
Environmental | Cloud Seeding | Enhances precipitation |
Case Study 1: Antibacterial Efficacy
A study conducted on this compound nanoparticles demonstrated their ability to induce ROS production leading to bacterial cell death. The research involved various bacterial strains, revealing that the nanoparticles effectively killed E. coli at low concentrations while exhibiting varying resistance levels among other strains .
Case Study 2: Solar Cell Efficiency
Research on this compound's application as a hole transport material in perovskite solar cells showed remarkable efficiency improvements, highlighting its potential role in advancing solar technology .
Case Study 3: Cloud Seeding Impact
Field studies on cloud seeding using this compound indicated successful enhancement of precipitation rates, demonstrating its practical application in environmental management strategies .
作用机制
Cuprous iodide can be compared with other copper(I) halides such as copper(I) chloride and copper(I) bromide:
Copper(I) Chloride (CuCl): Similar in structure and reactivity but has different solubility and stability properties.
Copper(I) Bromide (CuBr): Also similar in structure but has a different color and reactivity profile.
Uniqueness of this compound: this compound is unique due to its specific photophysical properties, making it suitable for applications in optoelectronics and as a hole transport material in solar cells .
相似化合物的比较
- Copper(I) Chloride (CuCl)
- Copper(I) Bromide (CuBr)
- Silver Iodide (AgI)
- Gold Monoiodide (AuI)
- Sodium Iodide (NaI)
生物活性
Cuprous iodide (CuI) is a compound that has garnered significant attention in recent years due to its diverse biological activities. This article explores the antibacterial, antiviral, and potential anticancer properties of CuI, supported by various studies and findings.
Antibacterial Activity
CuI exhibits notable antibacterial properties, particularly in nanoparticle form. A study demonstrated that copper iodide nanoparticles (CuI NPs), synthesized via co-precipitation, showed effective antibacterial activity against both Gram-positive and Gram-negative bacteria. The nanoparticles had an average size of 8 nm and were found to produce reactive oxygen species (ROS), which are crucial for their bactericidal action. The minimum inhibitory concentration (MIC) and minimum bactericidal concentration (MBC) values indicated that the strain DH5α was particularly sensitive to CuI NPs, with MIC and MBC values of 0.066 mg/ml and 0.083 mg/ml respectively, while Bacillus subtilis showed higher resistance with values of 0.15 mg/ml and 0.18 mg/ml .
Mechanism of Action:
- ROS Production: CuI NPs induce oxidative stress in bacterial cells by generating ROS which leads to DNA damage and membrane disruption.
- Membrane Damage: Atomic force microscopy (AFM) studies confirmed that CuI NPs cause significant damage to bacterial membranes, contributing to their antibacterial efficacy .
Antiviral Activity
Recent research has highlighted the effectiveness of CuI against SARS-CoV-2, the virus responsible for COVID-19. A study reported that CuI nanoparticles rapidly inactivated SARS-CoV-2 with high viral titers. The mechanism involved the generation of Cu+ ions, which exhibited strong virucidal activity through the production of ROS that damaged viral proteins and disrupted the viral genome .
Key Findings:
- Virucidal Mechanism: The destruction of viral proteins was primarily due to CuI-derived ROS, while lipid peroxidation induced by copper ions contributed to damage to the viral envelope.
- Application: CuI-doped films and fabrics demonstrated potential as materials for inactivating SARS-CoV-2 on high-touch surfaces, showcasing practical applications in infection control .
Anticancer Potential
The biological activity of iodine compounds, including CuI, has been investigated for their potential anticancer effects. Research indicates that iodine supplementation can exert antitumor effects by reducing cell proliferation and inducing apoptosis in various cancer cell lines. Specifically, studies have shown that iodide can decrease tumor growth in mouse models of prostate cancer .
Study Insights:
- Mechanism: Iodine influences cancer cell behavior by modulating pathways related to cell proliferation and apoptosis.
- Preclinical Models: In transgenic adenocarcinoma models, iodine uptake was analyzed alongside its effects on tumor growth and cellular mechanisms .
Summary Table of Biological Activities
常见问题
Basic Research Questions
Q. What are the optimal synthetic routes for producing high-purity cuprous iodide (CuI) in laboratory settings?
CuI is commonly synthesized via redox reactions between copper salts (e.g., CuSO₄) and iodide ions (I⁻) under acidic conditions. For high purity, stoichiometric control and inert atmospheres (e.g., N₂ or Ar) are critical to prevent oxidation to Cu(II) species. Post-synthesis purification via recrystallization in hydriodic acid (HI) or vacuum sublimation is recommended . Characterization using X-ray diffraction (XRD) and energy-dispersive X-ray spectroscopy (EDS) ensures phase purity and stoichiometric accuracy .
Q. How can researchers assess the purity and crystallinity of synthesized CuI?
Key methods include:
- XRD : To confirm crystallographic phase (e.g., γ-CuI vs. β-CuI) and detect impurities.
- SEM-EDS : For morphological analysis and elemental composition verification.
- ICP-MS : To quantify trace metal impurities.
- Thermogravimetric Analysis (TGA) : To evaluate thermal stability and decomposition pathways. Cross-referencing data from multiple techniques minimizes analytical bias .
Q. What role does CuI play in coordination chemistry, and how can its complexation behavior be studied?
CuI acts as a soft Lewis acid, forming complexes with ligands like amines or phosphines. To study coordination behavior:
- Use UV-Vis spectroscopy to monitor charge-transfer transitions.
- Employ NMR spectroscopy (¹H, ³¹P) for ligand binding analysis.
- Conduct single-crystal X-ray diffraction to resolve structural motifs. For example, CuI forms stable complexes with silver in electroplating applications, as noted in iodide-mediated coordination studies .
Advanced Research Questions
Q. How can experimentalists resolve contradictions in reported bandgap values of CuI (2.9–3.1 eV vs. 1.1–1.3 eV)?
Discrepancies arise from synthesis methods (e.g., solution vs. vapor-phase growth) and measurement techniques (UV-Vis vs. photoluminescence). To address this:
- Standardize synthesis protocols (e.g., solvent, temperature).
- Use spectroscopic ellipsometry for direct bandgap measurement.
- Compare results with computational models (e.g., DFT calculations) to account for excitonic effects .
Q. What methodologies are effective for studying CuI’s broadband luminescence in optoelectronic applications?
CuI’s self-trapped exciton (STE) emission (600–1000 nm) requires:
- Time-resolved photoluminescence (TRPL) : To probe emission lifetimes and recombination pathways.
- Temperature-dependent PL : To distinguish STE from defect-related emissions.
- Transient absorption spectroscopy : To map exciton dynamics. Recent studies on 3D [(Me)₂-DABCO]Cu₆I₈ frameworks highlight the role of structural distortion in red-NIR emission .
Q. How can researchers design experiments to evaluate CuI’s catalytic efficiency in cross-coupling reactions?
Key steps include:
- Kinetic studies : Monitor reaction rates under varying CuI concentrations.
- In situ XAFS/XANES : To track Cu oxidation state changes during catalysis.
- Control experiments : Compare with Cu(II) catalysts to isolate CuI-specific activity. Optimize ligand choice (e.g., phenanthroline) to stabilize active CuI intermediates .
Q. Data Analysis and Reproducibility
Q. What statistical approaches are recommended for analyzing inconsistent catalytic performance data in CuI-mediated reactions?
- Apply multivariate analysis (e.g., PCA) to identify variables (e.g., solvent polarity, ligand ratio) influencing outcomes.
- Use error-barred dose-response curves to quantify reproducibility.
- Cross-validate with machine learning models trained on reaction parameters .
Q. How can researchers ensure reproducibility in CuI thin-film deposition for photovoltaic studies?
- Document substrate pretreatment (e.g., UV-ozone cleaning).
- Standardize deposition parameters (e.g., spin-coating speed, annealing temperature).
- Use atomic force microscopy (AFM) to validate film homogeneity. Reference protocols from peer-reviewed studies on CuI as a hole-transport layer .
Q. Emerging Research Directions
Q. What advanced characterization techniques are critical for probing CuI’s role in perovskite solar cells?
- Impedance spectroscopy : To study charge-carrier transport.
- Kelvin probe force microscopy (KPFM) : To map work-function variations.
- In situ XRD : To monitor phase stability under operational conditions .
Q. How can computational modeling complement experimental studies of CuI’s defect chemistry?
属性
CAS 编号 |
1335-23-5 |
---|---|
分子式 |
CuI |
分子量 |
190.45 |
产品来源 |
United States |
体外研究产品的免责声明和信息
请注意,BenchChem 上展示的所有文章和产品信息仅供信息参考。 BenchChem 上可购买的产品专为体外研究设计,这些研究在生物体外进行。体外研究,源自拉丁语 "in glass",涉及在受控实验室环境中使用细胞或组织进行的实验。重要的是要注意,这些产品没有被归类为药物或药品,他们没有得到 FDA 的批准,用于预防、治疗或治愈任何医疗状况、疾病或疾病。我们必须强调,将这些产品以任何形式引入人类或动物的身体都是法律严格禁止的。遵守这些指南对确保研究和实验的法律和道德标准的符合性至关重要。