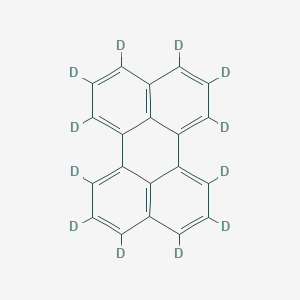
苝-D12
概述
描述
Perylene-D12 is a deuterated form of perylene, a polycyclic aromatic hydrocarbon. The chemical formula for Perylene-D12 is C20D12, and it has a molecular weight of 264.3832 . Deuterium, a stable isotope of hydrogen, replaces all hydrogen atoms in the perylene molecule, making Perylene-D12 particularly useful in various scientific applications, especially in spectroscopy and mass spectrometry.
科学研究应用
Perylene-D12 has a wide range of applications in scientific research:
Chemistry: Used as an internal standard in mass spectrometry due to its stable isotopic composition.
Biology: Employed as a fluorescent probe in cell membrane studies.
Medicine: Utilized in the development of diagnostic imaging agents.
作用机制
Target of Action
Perylene-D12 is a deuterium-labeled version of Perylene . The primary targets of Perylene-D12 are not explicitly mentioned in the available literature. More research is needed to identify the specific molecular targets of Perylene-D12.
Mode of Action
It’s known that perylene-d12 interacts with its environment through various forces such as chemical attraction, pauli repulsion, and van der waals (vdw) interaction .
Biochemical Pathways
One study mentions the interaction of a perylene derivative (perylene-tetracarboxylic dianhydride) with au(111), which could suggest potential interactions with metal ions or surfaces .
Result of Action
It’s known that perylene-d12 is a stable isotope-labeled compound , which suggests it could be used in research to track and quantify its distribution and effects in biological systems.
Action Environment
The action of Perylene-D12 can be influenced by environmental factors. For instance, the stability of a perylene derivative was studied on a Au(111) surface , suggesting that the surface and environmental conditions could impact the action and stability of Perylene-D12.
生化分析
Biochemical Properties
Perylene-D12 plays a role in biochemical reactions, particularly as an acceptor for pristine C70, used as a sensitizer for efficient triplet-triplet annihilation upconversion . This process has shown improved stability under continuous laser irradiation compared to the benchmark Pt (II)-ocetaethylprphyrin .
Cellular Effects
These conjugates also enhanced cell viabilities in cryopreservation effects .
Molecular Mechanism
Perylene derivatives have been used as promising scaffolds for the design of small molecule/polymer-based chromogenic and fluorogenic reaction-based probes due to their strong absorption combined with high fluorescence quantum yield in organic solvents, low reduction potential, good electron-acceptor properties, and broad color range properties .
Metabolic Pathways
It is known that the microbial transformation of PAHs under aerobic conditions typically starts with the hydroxylation of an aromatic ring via a ring hydroxylating dioxygenase .
准备方法
Synthetic Routes and Reaction Conditions: Perylene-D12 is synthesized through a multi-step process involving the deuteration of perylene. The process typically starts with the preparation of perylene, followed by the exchange of hydrogen atoms with deuterium. This can be achieved using deuterium gas (D2) under high pressure and temperature conditions .
Industrial Production Methods: Industrial production of Perylene-D12 involves the use of specialized reactors that can handle high-pressure deuterium gas. The process is carefully controlled to ensure complete deuteration of the perylene molecule. The final product is purified using techniques such as recrystallization and chromatography to achieve high isotopic purity .
化学反应分析
Types of Reactions: Perylene-D12, like its non-deuterated counterpart, undergoes various chemical reactions, including:
Oxidation: Perylene-D12 can be oxidized to form perylenequinone derivatives.
Reduction: It can be reduced by alkali metals to form radical anions and dianions.
Substitution: Electrophilic substitution reactions can occur, where deuterium atoms are replaced by other substituents.
Common Reagents and Conditions:
Oxidation: Common oxidizing agents include potassium permanganate and chromium trioxide.
Reduction: Alkali metals such as sodium or potassium in the presence of solvents like tetrahydrofuran.
Substitution: Electrophilic reagents such as halogens or nitro compounds under acidic conditions.
Major Products:
Oxidation: Perylenequinone derivatives.
Reduction: Radical anions and dianions.
Substitution: Various substituted perylene derivatives depending on the electrophile used.
相似化合物的比较
Perylene: The non-deuterated form of Perylene-D12, with a chemical formula of C20H12.
Phenanthrene-D10: Another deuterated polycyclic aromatic hydrocarbon used in similar applications.
Naphthalene-D8: A smaller deuterated polycyclic aromatic hydrocarbon used as an internal standard in various analytical techniques.
Uniqueness of Perylene-D12: Perylene-D12 is unique due to its complete deuteration, which provides distinct advantages in spectroscopic and mass spectrometric analyses. Its high isotopic purity and stability make it an ideal internal standard and fluorescent probe, distinguishing it from other similar compounds .
属性
IUPAC Name |
1,2,3,4,5,6,7,8,9,10,11,12-dodecadeuterioperylene | |
---|---|---|
Source | PubChem | |
URL | https://pubchem.ncbi.nlm.nih.gov | |
Description | Data deposited in or computed by PubChem | |
InChI |
InChI=1S/C20H12/c1-5-13-6-2-11-17-18-12-4-8-14-7-3-10-16(20(14)18)15(9-1)19(13)17/h1-12H/i1D,2D,3D,4D,5D,6D,7D,8D,9D,10D,11D,12D | |
Source | PubChem | |
URL | https://pubchem.ncbi.nlm.nih.gov | |
Description | Data deposited in or computed by PubChem | |
InChI Key |
CSHWQDPOILHKBI-AQZSQYOVSA-N | |
Source | PubChem | |
URL | https://pubchem.ncbi.nlm.nih.gov | |
Description | Data deposited in or computed by PubChem | |
Canonical SMILES |
C1=CC2=C3C(=C1)C4=CC=CC5=C4C(=CC=C5)C3=CC=C2 | |
Source | PubChem | |
URL | https://pubchem.ncbi.nlm.nih.gov | |
Description | Data deposited in or computed by PubChem | |
Isomeric SMILES |
[2H]C1=C(C2=C3C(=C1[2H])C4=C(C(=C(C5=C4C(=C(C(=C5[2H])[2H])[2H])C3=C(C(=C2[2H])[2H])[2H])[2H])[2H])[2H])[2H] | |
Source | PubChem | |
URL | https://pubchem.ncbi.nlm.nih.gov | |
Description | Data deposited in or computed by PubChem | |
Molecular Formula |
C20H12 | |
Source | PubChem | |
URL | https://pubchem.ncbi.nlm.nih.gov | |
Description | Data deposited in or computed by PubChem | |
DSSTOX Substance ID |
DTXSID60934397 | |
Record name | (~2~H_12_)Perylene | |
Source | EPA DSSTox | |
URL | https://comptox.epa.gov/dashboard/DTXSID60934397 | |
Description | DSSTox provides a high quality public chemistry resource for supporting improved predictive toxicology. | |
Molecular Weight |
264.4 g/mol | |
Source | PubChem | |
URL | https://pubchem.ncbi.nlm.nih.gov | |
Description | Data deposited in or computed by PubChem | |
Physical Description |
Solid; [Sigma-Aldrich MSDS] | |
Record name | Perylene-d12 | |
Source | Haz-Map, Information on Hazardous Chemicals and Occupational Diseases | |
URL | https://haz-map.com/Agents/20540 | |
Description | Haz-Map® is an occupational health database designed for health and safety professionals and for consumers seeking information about the adverse effects of workplace exposures to chemical and biological agents. | |
Explanation | Copyright (c) 2022 Haz-Map(R). All rights reserved. Unless otherwise indicated, all materials from Haz-Map are copyrighted by Haz-Map(R). No part of these materials, either text or image may be used for any purpose other than for personal use. Therefore, reproduction, modification, storage in a retrieval system or retransmission, in any form or by any means, electronic, mechanical or otherwise, for reasons other than personal use, is strictly prohibited without prior written permission. | |
CAS No. |
1520-96-3 | |
Record name | Perylene-d12 | |
Source | ChemIDplus | |
URL | https://pubchem.ncbi.nlm.nih.gov/substance/?source=chemidplus&sourceid=0001520963 | |
Description | ChemIDplus is a free, web search system that provides access to the structure and nomenclature authority files used for the identification of chemical substances cited in National Library of Medicine (NLM) databases, including the TOXNET system. | |
Record name | (~2~H_12_)Perylene | |
Source | EPA DSSTox | |
URL | https://comptox.epa.gov/dashboard/DTXSID60934397 | |
Description | DSSTox provides a high quality public chemistry resource for supporting improved predictive toxicology. | |
Record name | 1520-96-3 | |
Source | European Chemicals Agency (ECHA) | |
URL | https://echa.europa.eu/information-on-chemicals | |
Description | The European Chemicals Agency (ECHA) is an agency of the European Union which is the driving force among regulatory authorities in implementing the EU's groundbreaking chemicals legislation for the benefit of human health and the environment as well as for innovation and competitiveness. | |
Explanation | Use of the information, documents and data from the ECHA website is subject to the terms and conditions of this Legal Notice, and subject to other binding limitations provided for under applicable law, the information, documents and data made available on the ECHA website may be reproduced, distributed and/or used, totally or in part, for non-commercial purposes provided that ECHA is acknowledged as the source: "Source: European Chemicals Agency, http://echa.europa.eu/". Such acknowledgement must be included in each copy of the material. ECHA permits and encourages organisations and individuals to create links to the ECHA website under the following cumulative conditions: Links can only be made to webpages that provide a link to the Legal Notice page. | |
Synthesis routes and methods I
Procedure details
Synthesis routes and methods II
Procedure details
Synthesis routes and methods III
Procedure details
Synthesis routes and methods IV
Procedure details
Synthesis routes and methods V
Procedure details
Retrosynthesis Analysis
AI-Powered Synthesis Planning: Our tool employs the Template_relevance Pistachio, Template_relevance Bkms_metabolic, Template_relevance Pistachio_ringbreaker, Template_relevance Reaxys, Template_relevance Reaxys_biocatalysis model, leveraging a vast database of chemical reactions to predict feasible synthetic routes.
One-Step Synthesis Focus: Specifically designed for one-step synthesis, it provides concise and direct routes for your target compounds, streamlining the synthesis process.
Accurate Predictions: Utilizing the extensive PISTACHIO, BKMS_METABOLIC, PISTACHIO_RINGBREAKER, REAXYS, REAXYS_BIOCATALYSIS database, our tool offers high-accuracy predictions, reflecting the latest in chemical research and data.
Strategy Settings
Precursor scoring | Relevance Heuristic |
---|---|
Min. plausibility | 0.01 |
Model | Template_relevance |
Template Set | Pistachio/Bkms_metabolic/Pistachio_ringbreaker/Reaxys/Reaxys_biocatalysis |
Top-N result to add to graph | 6 |
Feasible Synthetic Routes
Q1: Why is Perylene-D12 frequently used as an internal standard in PAH analysis?
A1: Perylene-D12's chemical structure closely resembles that of target PAHs, leading to similar chromatographic behavior. The twelve deuterium atoms increase its mass, allowing clear distinction from native perylene and other PAHs during mass spectrometric detection [, , , ].
Q2: Can you provide examples of specific applications of Perylene-D12 as an internal standard?
A2: Perylene-D12 has been successfully employed to quantify PAHs in diverse matrices like air samples [, ], river water and suspended particles [], groundwater [], edible oils [], fish samples [], and beers [].
Q3: What are the advantages of using multiple internal standards in PAH analysis, and how is Perylene-D12 incorporated?
A3: Employing multiple internal standards, including Perylene-D12 alongside others like acenaphthene-d10, chrysene-d12, and phenanthrene-d10, enhances the accuracy and precision of PAH quantification by accounting for variations in extraction efficiency and instrument response across a wider range of analyte properties [, , , ].
Q4: How does the use of Perylene-D12 contribute to accurate quantification in GC-MS analysis of PAHs?
A4: The "internal standard method" used in conjunction with Perylene-D12 involves adding a known amount of the standard to the sample before extraction and analysis. By comparing the peak area ratio of the target PAH to Perylene-D12, variations in extraction recovery and instrument response can be corrected, leading to more accurate concentration determination [, , ].
Q5: What is the molecular formula and weight of Perylene-D12?
A5: The molecular formula of Perylene-D12 is C20D12. Its molecular weight is 264.38 g/mol.
Q6: What spectroscopic techniques are used to characterize Perylene-D12?
A6: Gas chromatography-mass spectrometry (GC-MS) is the primary technique used for detection and quantification of Perylene-D12 in analytical applications [, , , , , , ]. Additionally, research on its phonon dispersion curves using coherent inelastic neutron scattering and Raman scattering has been conducted [].
Q7: Does the use of Perylene-D12 raise any environmental concerns?
A7: While Perylene-D12 itself has limited environmental impact due to its use in trace amounts, it's important to note that PAHs, which it helps to quantify, are a significant environmental concern due to their persistence, bioaccumulation, and toxicity [, , ].
Q8: Are there any alternatives to Perylene-D12 as an internal standard for PAH analysis?
A8: While other deuterated PAHs like naphthalene-d8, acenaphthene-d10, phenanthrene-d10, and chrysene-d12 are also used [, , , ], the choice of internal standard depends on the specific PAHs being analyzed and the matrix complexity.
Q9: How can computational chemistry contribute to research on Perylene-D12 and PAH analysis?
A9: Computational methods can be used to predict the fragmentation patterns of Perylene-D12 and other PAHs in mass spectrometry, aiding in method development and optimization. Additionally, simulations can help understand the interactions between PAHs and different extraction phases, contributing to improved sample preparation techniques [].
Q10: What are potential future research directions related to Perylene-D12 and PAH analysis?
A10: Future research could explore the use of Perylene-D12 in emerging analytical techniques like high-resolution mass spectrometry (HRMS) for more precise and sensitive PAH quantification. Additionally, developing greener and more sustainable sample preparation methods that minimize solvent use while maintaining analytical performance is an important area for exploration [].
体外研究产品的免责声明和信息
请注意,BenchChem 上展示的所有文章和产品信息仅供信息参考。 BenchChem 上可购买的产品专为体外研究设计,这些研究在生物体外进行。体外研究,源自拉丁语 "in glass",涉及在受控实验室环境中使用细胞或组织进行的实验。重要的是要注意,这些产品没有被归类为药物或药品,他们没有得到 FDA 的批准,用于预防、治疗或治愈任何医疗状况、疾病或疾病。我们必须强调,将这些产品以任何形式引入人类或动物的身体都是法律严格禁止的。遵守这些指南对确保研究和实验的法律和道德标准的符合性至关重要。