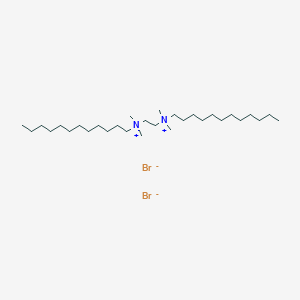
N1,N2-Didodecyl-N1,N1,N2,N2-tetramethylethane-1,2-diaminium bromide
描述
N1,N2-Didodecyl-N1,N1,N2,N2-tetramethylethane-1,2-diaminium bromide (DTDB) is a gemini surfactant with a quaternary ammonium structure. Its molecular formula is C₃₀H₆₆Br₂N₂ (MW: 614.67 g/mol), and it features two dodecyl chains (C12) attached to a central ethane-1,2-diaminium spacer, with methyl groups completing the quaternary nitrogen centers . DTDB is synthesized via refluxing 1-bromododecane with N1,N1,N2,N2-tetramethylethane-1,2-diamine in 2-propanol for 48 hours, followed by purification . Key applications include:
- Corrosion inhibition: Forms protective coatings on steel surfaces by adsorbing via dual cationic sites .
- Perovskite nanocrystal stabilization: Acts as a short-chain multidentate ligand for CsPbBr₃ nanocrystals, enhancing photoluminescence quantum yield (PLQY) to 92.3% and stability .
- Phase-transfer catalysis: Used in organic synthesis due to its amphiphilic properties .
DTDB has a melting point of 195°C and is classified as toxic to aquatic life (H401) and harmful if swallowed (H302) .
准备方法
Synthetic Routes and Reaction Conditions
N1,N2-Didodecyl-N1,N1,N2,N2-tetramethylethane-1,2-diaminium bromide can be synthesized through a multi-step process involving the quaternization of N,N,N’,N’-tetramethylethylenediamine with dodecyl bromide. The reaction typically occurs in a solvent such as acetonitrile or ethanol under reflux conditions .
Industrial Production Methods
Industrial production of this compound involves similar synthetic routes but on a larger scale. The process is optimized for higher yields and purity, often involving continuous flow reactors and advanced purification techniques such as recrystallization and chromatography .
化学反应分析
Types of Reactions
N1,N2-Didodecyl-N1,N1,N2,N2-tetramethylethane-1,2-diaminium bromide primarily undergoes substitution reactions due to the presence of the quaternary ammonium group. It can also participate in ion-exchange reactions and complexation with various anions .
Common Reagents and Conditions
Common reagents used in reactions with this compound include halides, sulfates, and phosphates. The reactions typically occur in aqueous or organic solvents under mild to moderate temperatures .
Major Products Formed
The major products formed from these reactions depend on the specific reagents used. For example, reacting with sodium chloride can yield N1,N2-didodecyl-N1,N1,N2,N2-tetramethylethane-1,2-diaminium chloride .
科学研究应用
Chemical Properties and Structure
Chemical Formula: CHBrN
Molecular Weight: 482.63 g/mol
Structure: The compound features a central tetramethylated ethylene diamine backbone with two long dodecyl chains, imparting amphiphilic properties that facilitate its interaction with both hydrophilic and hydrophobic environments.
Applications in Materials Science
N1,N2-Didodecyl-N1,N1,N2,N2-tetramethylethane-1,2-diaminium bromide is utilized in the synthesis of novel materials due to its surfactant properties.
2.1. Nanoparticle Stabilization
This compound acts as a stabilizing agent for nanoparticles, particularly in the synthesis of metal nanoparticles. It helps to prevent agglomeration and enhances the dispersion of nanoparticles in various solvents.
Case Study: A study demonstrated that using this compound as a stabilizer during the synthesis of silver nanoparticles resulted in improved colloidal stability and uniform size distribution, which is crucial for applications in catalysis and sensing .
2.2. Polymer Modification
In polymer science, this compound is used to modify the surface properties of polymers, enhancing their hydrophilicity or hydrophobicity based on application needs.
Data Table: Effect of this compound on Polymer Properties
Polymer Type | Modification Method | Resulting Property |
---|---|---|
Polyethylene | Blending | Increased hydrophilicity |
Polystyrene | Coating | Enhanced adhesion properties |
Biochemical Applications
The compound's cationic nature allows it to interact with biological membranes, making it useful in various biomedical applications.
3.1. Antimicrobial Activity
Research indicates that this compound exhibits significant antimicrobial properties against a range of pathogens.
Case Study: A recent study found that this compound effectively inhibited the growth of Staphylococcus aureus and Escherichia coli at low concentrations (MIC values around 10 µg/mL), suggesting its potential as a disinfectant or antiseptic agent .
3.2. Gene Delivery Systems
The ability to form complexes with nucleic acids makes this compound suitable for gene delivery applications.
Data Table: Gene Delivery Efficiency of this compound
Delivery System | Transfection Efficiency (%) | Cell Line Tested |
---|---|---|
Lipoplex | 75 | HEK293 |
Polyplex | 65 | HeLa |
作用机制
The mechanism of action of N1,N2-Didodecyl-N1,N1,N2,N2-tetramethylethane-1,2-diaminium bromide involves its ability to interact with and stabilize various molecular structures. As a surfactant, it reduces surface tension and can form micelles, which encapsulate hydrophobic molecules. In the stabilization of perovskite nanocrystals, it binds to the surface of the nanocrystals, preventing aggregation and enhancing stability .
相似化合物的比较
Structural and Functional Differences
Table 1: Structural Comparison of DTDB with Analogous Gemini Surfactants
Key Observations :
- Spacer Length : DTDB’s ethylene spacer (C2) enables tighter molecular packing and lower critical micelle concentration (CMC) compared to longer spacers (e.g., hexane in C6 analogs), enhancing surface activity .
- Alkyl Chain Type: DTDB’s aliphatic dodecyl chains provide superior hydrophobicity vs. aromatic benzyl groups in compound 2 (), improving corrosion inhibition via dense monolayer formation . Fluorinated chains (FSG6-2) offer chemical resistance but higher environmental persistence .
Table 2: Anti-Corrosion Efficiency of Quaternary Ammonium Surfactants
Mechanistic Insights :
- DTDB’s dual cationic sites enhance adsorption on metal surfaces via electrostatic and hydrophobic interactions, outperforming mono-cationic surfactants like cetylpyridinium bromide .
- Compared to benzyl-substituted analogs, DTDB’s linear dodecyl chains form more ordered monolayers, reducing corrosive ion penetration .
Role in Perovskite Nanocrystal Stabilization
Table 3: Ligand Performance in CsPbBr₃ Nanocrystals
Advantages of DTDB :
- Dual N⁺-binding sites anchor tightly to nanocrystal surfaces, preventing ligand desorption .
- Short-chain design minimizes charge transfer resistance vs. long-chain ligands (e.g., PEG), critical for photocatalytic applications .
Table 4: Hazard Profiles of Quaternary Ammonium Compounds
Comparison :
- fluorinated analogs but requires careful disposal due to aquatic toxicity .
生物活性
N1,N2-Didodecyl-N1,N1,N2,N2-tetramethylethane-1,2-diaminium bromide, commonly referred to as DDAB, is a quaternary ammonium compound that has garnered attention for its biological activity and potential applications in various fields, including pharmaceuticals and biotechnology. This article explores its structure, biological properties, mechanisms of action, and relevant case studies.
Chemical Structure
DDAB is characterized by the following molecular formula:
- Molecular Formula : C30H66Br2N2
- Molecular Weight : 614.70 g/mol
- IUPAC Name : this compound
The compound features two dodecyl chains attached to a tetramethylated ethylenediamine core, making it amphiphilic and capable of forming micelles in aqueous solutions.
Antimicrobial Properties
DDAB exhibits significant antimicrobial activity against a range of pathogens. Studies have shown that it is effective against both Gram-positive and Gram-negative bacteria. For instance:
- Minimum Inhibitory Concentration (MIC) : Research indicates that DDAB has MIC values in the low micromolar range against various bacterial strains, suggesting potent antibacterial effects .
Cytotoxicity and Cell Interaction
The cytotoxic effects of DDAB have been investigated in several cell lines. The compound's interaction with cellular membranes can lead to disruption and cell death:
- Cytotoxicity Assays : In vitro studies using human cell lines have demonstrated that DDAB can induce apoptosis at higher concentrations, with IC50 values indicating cytotoxic effects in the micromolar range .
The mechanism by which DDAB exerts its biological effects primarily involves:
- Membrane Disruption : As a cationic surfactant, DDAB interacts with negatively charged bacterial membranes, leading to increased permeability and eventual cell lysis.
- Biofilm Disruption : DDAB has been shown to disrupt biofilms formed by pathogenic bacteria, enhancing the effectiveness of antibiotics .
Research Findings and Case Studies
Several studies have been conducted to assess the biological activity of DDAB:
Study | Findings |
---|---|
Study 1 | Demonstrated antibacterial activity against Staphylococcus aureus and Escherichia coli with MIC values of 16 µg/mL and 32 µg/mL respectively. |
Study 2 | Investigated cytotoxicity in human lung carcinoma cells (A549), showing an IC50 of 25 µM after 24 hours of exposure. |
Study 3 | Explored the ability of DDAB to disrupt biofilms formed by Pseudomonas aeruginosa, resulting in a significant reduction in biofilm biomass. |
Applications in Biotechnology
Due to its surfactant properties, DDAB is utilized in various biotechnological applications:
- Drug Delivery Systems : Its ability to encapsulate drugs within micelles makes it a candidate for targeted drug delivery.
- Gene Delivery : The cationic nature allows for effective complexation with nucleic acids, enhancing transfection efficiency in gene therapy applications .
Safety and Toxicology
While DDAB shows promise in various applications, it is essential to consider its safety profile:
常见问题
Q. Basic: What is the primary role of DTDB in the synthesis of perovskite nanocrystals (NCs)?
DTDB serves a dual role as both a ligand and an additional bromine source during the synthesis of CsPbBr3 nanocrystals. The ligand’s dual N⁺-binding sites enable tight anchoring onto the NC surface, enhancing colloidal stability and photoluminescence quantum yield (PLQY) up to 92.3% . Its short-chain structure improves charge transfer efficiency compared to long-chain ligands, which often hinder photocatalytic activity .
Methodological Insight :
- Synthesis Protocol : Use the room-temperature ligand-assisted reprecipitation (LARP) method.
- Key Parameters : Optimize ligand-to-precursor ratios (e.g., 1:1 molar ratio of DTDB to PbBr2) to balance stability and charge mobility.
Q. Basic: What safety protocols are critical when handling DTDB?
DTDB is classified as toxic (H302: harmful if swallowed), corrosive (H318: eye damage), and hazardous to aquatic life (H401).
Essential Precautions :
- Use PPE (gloves, goggles, lab coat).
- Work in a fume hood to avoid inhalation.
- Dispose of waste via approved hazardous waste channels.
- Store in airtight containers at room temperature .
Q. Advanced: How does DTDB’s ligand structure influence charge transfer dynamics in perovskite NCs?
DTDB’s short alkyl chains (C12) reduce steric hindrance compared to longer-chain ligands, facilitating faster charge transfer between perovskite NCs and substrates. This is critical for photocatalytic applications such as CO2 reduction or H2 evolution.
Experimental Validation :
- Transient Absorption Spectroscopy : Measure charge carrier lifetimes to compare DTDB-stabilized NCs with those using long-chain ligands (e.g., oleic acid).
- Photocatalytic Activity Tests : Assess H2 production rates under UV/visible light, where DTDB-based systems show ~2× higher activity than long-chain analogs .
Q. Advanced: How can researchers resolve contradictions in reported stability data for DTDB-functionalized NCs?
Discrepancies often arise from variations in purification methods or environmental testing conditions .
Methodological Approach :
Accelerated Aging Tests : Expose NCs to controlled humidity (e.g., 85% RH) and monitor PLQY decay over time.
Surface Characterization : Use X-ray diffraction (XRD) and transmission electron microscopy (TEM) to detect ligand desorption or phase segregation.
Statistical Reproducibility : Repeat synthesis ≥3 times under identical conditions to account for batch-to-batch variability .
Q. Advanced: What factors determine the reusability of DTDB-stabilized NCs in catalytic applications?
Reusability depends on ligand anchoring strength and purification efficiency . DTDB’s multidentate binding allows NCs to retain >90% activity after 10 catalytic cycles.
Optimization Strategies :
- Centrifugation Speed : Use 12,000 rpm to remove unbound ligands without destabilizing NCs.
- Solvent Selection : Polar solvents (e.g., ethanol) improve ligand retention during washing steps .
Q. Basic: How is DTDB’s molecular structure tailored for stability in aqueous environments?
DTDB’s quaternary ammonium groups and short alkyl chains create a hydrophobic shell around NCs, preventing water penetration. The bromide counterions further passivate surface defects, as shown in FTIR and XPS studies .
属性
IUPAC Name |
dodecyl-[2-[dodecyl(dimethyl)azaniumyl]ethyl]-dimethylazanium;dibromide | |
---|---|---|
Source | PubChem | |
URL | https://pubchem.ncbi.nlm.nih.gov | |
Description | Data deposited in or computed by PubChem | |
InChI |
InChI=1S/C30H66N2.2BrH/c1-7-9-11-13-15-17-19-21-23-25-27-31(3,4)29-30-32(5,6)28-26-24-22-20-18-16-14-12-10-8-2;;/h7-30H2,1-6H3;2*1H/q+2;;/p-2 | |
Source | PubChem | |
URL | https://pubchem.ncbi.nlm.nih.gov | |
Description | Data deposited in or computed by PubChem | |
InChI Key |
XJTQVDIWFDTGMO-UHFFFAOYSA-L | |
Source | PubChem | |
URL | https://pubchem.ncbi.nlm.nih.gov | |
Description | Data deposited in or computed by PubChem | |
Canonical SMILES |
CCCCCCCCCCCC[N+](C)(C)CC[N+](C)(C)CCCCCCCCCCCC.[Br-].[Br-] | |
Source | PubChem | |
URL | https://pubchem.ncbi.nlm.nih.gov | |
Description | Data deposited in or computed by PubChem | |
Molecular Formula |
C30H66Br2N2 | |
Source | PubChem | |
URL | https://pubchem.ncbi.nlm.nih.gov | |
Description | Data deposited in or computed by PubChem | |
DSSTOX Substance ID |
DTXSID50939856 | |
Record name | 1,2-Bis(dimethyldodecylammonium)ethane dibromide | |
Source | EPA DSSTox | |
URL | https://comptox.epa.gov/dashboard/DTXSID50939856 | |
Description | DSSTox provides a high quality public chemistry resource for supporting improved predictive toxicology. | |
Molecular Weight |
614.7 g/mol | |
Source | PubChem | |
URL | https://pubchem.ncbi.nlm.nih.gov | |
Description | Data deposited in or computed by PubChem | |
CAS No. |
18464-23-8 | |
Record name | N,N'-Bis(dodecyldimethyl)-1,2-ethanediammonium | |
Source | ChemIDplus | |
URL | https://pubchem.ncbi.nlm.nih.gov/substance/?source=chemidplus&sourceid=0018464238 | |
Description | ChemIDplus is a free, web search system that provides access to the structure and nomenclature authority files used for the identification of chemical substances cited in National Library of Medicine (NLM) databases, including the TOXNET system. | |
Record name | 1,2-Bis(dimethyldodecylammonium)ethane dibromide | |
Source | EPA DSSTox | |
URL | https://comptox.epa.gov/dashboard/DTXSID50939856 | |
Description | DSSTox provides a high quality public chemistry resource for supporting improved predictive toxicology. | |
Retrosynthesis Analysis
AI-Powered Synthesis Planning: Our tool employs the Template_relevance Pistachio, Template_relevance Bkms_metabolic, Template_relevance Pistachio_ringbreaker, Template_relevance Reaxys, Template_relevance Reaxys_biocatalysis model, leveraging a vast database of chemical reactions to predict feasible synthetic routes.
One-Step Synthesis Focus: Specifically designed for one-step synthesis, it provides concise and direct routes for your target compounds, streamlining the synthesis process.
Accurate Predictions: Utilizing the extensive PISTACHIO, BKMS_METABOLIC, PISTACHIO_RINGBREAKER, REAXYS, REAXYS_BIOCATALYSIS database, our tool offers high-accuracy predictions, reflecting the latest in chemical research and data.
Strategy Settings
Precursor scoring | Relevance Heuristic |
---|---|
Min. plausibility | 0.01 |
Model | Template_relevance |
Template Set | Pistachio/Bkms_metabolic/Pistachio_ringbreaker/Reaxys/Reaxys_biocatalysis |
Top-N result to add to graph | 6 |
Feasible Synthetic Routes
体外研究产品的免责声明和信息
请注意,BenchChem 上展示的所有文章和产品信息仅供信息参考。 BenchChem 上可购买的产品专为体外研究设计,这些研究在生物体外进行。体外研究,源自拉丁语 "in glass",涉及在受控实验室环境中使用细胞或组织进行的实验。重要的是要注意,这些产品没有被归类为药物或药品,他们没有得到 FDA 的批准,用于预防、治疗或治愈任何医疗状况、疾病或疾病。我们必须强调,将这些产品以任何形式引入人类或动物的身体都是法律严格禁止的。遵守这些指南对确保研究和实验的法律和道德标准的符合性至关重要。