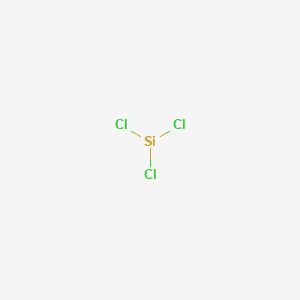
三氯硅基
描述
科学研究应用
Trichlorosilane has numerous applications in scientific research and industry:
Semiconductor Industry: It is a key precursor in the production of ultrapure silicon for semiconductor devices.
Surface Modification: Used to create self-assembled monolayers on various surfaces, enhancing properties such as hydrophobicity and adhesion.
Organic Synthesis: Employed as a reagent in the synthesis of various organosilicon compounds.
Polymer Coatings: Utilized in the production of silane-based polymer coatings for improved durability and resistance.
作用机制
Target of Action
Trichlorosilyl, also known as Trichlorosilyl radical or Silane, trichloro-, primarily targets p-block elements . These elements include Boron (B), Carbon ©, Silicon (Si), Germanium (Ge), Phosphorus (P), and Sulfur (S) . The compound forms anions with these elements, which are stabilized solely by trichlorosilyl groups .
Mode of Action
Trichlorosilyl interacts with its targets through the formation of anions . These anions, denoted as [E(SiCl3)n], exhibit high reactivity due to the presence of trichlorosilyl groups . They have been used in substitution reactions, as superacids, and weakly coordinating anions . This diverse reactivity makes these anions useful as reagents in a number of transformations .
Biochemical Pathways
It is known that the compound can introduce the central atom e into new compounds , suggesting that it may influence various biochemical pathways.
Pharmacokinetics
Computational platforms like admetlab and admetSAR can be used to predict these properties.
Result of Action
The molecular and cellular effects of Trichlorosilyl’s action are largely dependent on its high reactivity . It can introduce the central atom E into new compounds , potentially altering their structure and function.
准备方法
Synthetic Routes and Reaction Conditions
Trichlorosilane is primarily produced by treating powdered metallurgical grade silicon with hydrogen chloride gas at approximately 300°C. This reaction yields trichlorosilane and hydrogen gas : [ \text{Si} + 3 \text{HCl} \rightarrow \text{HCl}_3\text{Si} + \text{H}_2 ]
Another method involves the reaction of silicon tetrachloride with hydrogen gas : [ \text{SiCl}_4 + \text{H}_2 \rightarrow \text{HCl}_3\text{Si} + \text{HCl} ]
Industrial Production Methods
In industrial settings, trichlorosilane is produced in large quantities using the above methods. The process involves the chlorination of silicon, followed by purification through distillation to separate trichlorosilane from byproducts such as silicon tetrachloride and hexachlorodisilane .
化学反应分析
Types of Reactions
Trichlorosilane undergoes various chemical reactions, including:
Hydrolysis: In the presence of water, trichlorosilane hydrolyzes to form siloxane polymers and hydrochloric acid.
Hydrosilylation: Trichlorosilane reacts with alkenes in the presence of a catalyst to form organosilicon compounds.
Reduction: Trichlorosilane can reduce aldehydes to alcohols and imines to amines.
Common Reagents and Conditions
Hydrolysis: Water is the primary reagent, and the reaction occurs readily at room temperature.
Hydrosilylation: Catalysts such as platinum or rhodium are used, and the reaction typically occurs at elevated temperatures.
Reduction: Dimethylformamide can be used as a reagent for the reduction reactions.
Major Products
Hydrolysis: Siloxane polymers and hydrochloric acid.
Hydrosilylation: Organosilicon compounds such as octadecyltrichlorosilane.
Reduction: Alcohols and amines.
相似化合物的比较
Trichlorosilane is similar to other chlorosilanes such as dichlorosilane (H₂SiCl₂) and silicon tetrachloride (SiCl₄). trichlorosilane is unique due to its specific reactivity and applications:
Dichlorosilane: Less reactive and primarily used in the production of silicon-based polymers.
Silicon Tetrachloride: Used in the production of optical fibers and as a precursor to other silicon compounds.
生物活性
Trichlorosilyl compounds, particularly trichlorosilane (HSiCl₃), are notable in various chemical reactions and applications, including organic synthesis and materials science. This article explores the biological activity of trichlorosilyl compounds, focusing on their mechanisms, applications in catalysis, and potential health impacts based on recent research findings.
Overview of Trichlorosilyl Compounds
Trichlorosilyl compounds are organosilicon compounds characterized by the presence of silicon bonded to three chlorine atoms. These compounds serve as versatile intermediates in organic synthesis, particularly in the formation of siloxanes and silanes. Their reactivity is primarily due to the electrophilic nature of the silicon atom, which can participate in various nucleophilic attacks.
Mechanisms of Biological Activity
-
Catalytic Role in Organic Reactions :
- Trichlorosilyl triflate has been shown to facilitate enantioselective aldol reactions when used with chiral Lewis base catalysts. This reaction is significant for synthesizing complex organic molecules with high stereochemical purity .
- The mechanism involves the activation of carbonyl groups, allowing for the formation of trichlorosilyl enolates that participate in subsequent reactions, contributing to the synthesis of biologically active compounds .
-
Potential Antimicrobial Properties :
- Some studies suggest that trichlorosilyl compounds may exhibit antimicrobial properties when used as surface coatings or in formulations aimed at reducing microbial contamination . The efficacy of these compounds is attributed to their ability to form stable siloxane networks that can inhibit microbial growth.
Table 1: Summary of Research Findings on Trichlorosilyl Compounds
Health Implications
While trichlorosilyl compounds are valuable in synthetic chemistry, their biological activity raises concerns regarding occupational exposure and potential health risks. A study indicated a correlation between high exposure levels to trichloroethylene (TCE), a related compound, and an increased risk of non-Hodgkin lymphoma . This finding underscores the importance of safety measures when handling chlorinated solvents and related chemicals.
属性
InChI |
InChI=1S/Cl3Si/c1-4(2)3 | |
---|---|---|
Source | PubChem | |
URL | https://pubchem.ncbi.nlm.nih.gov | |
Description | Data deposited in or computed by PubChem | |
InChI Key |
PPDADIYYMSXQJK-UHFFFAOYSA-N | |
Source | PubChem | |
URL | https://pubchem.ncbi.nlm.nih.gov | |
Description | Data deposited in or computed by PubChem | |
Canonical SMILES |
[Si](Cl)(Cl)Cl | |
Source | PubChem | |
URL | https://pubchem.ncbi.nlm.nih.gov | |
Description | Data deposited in or computed by PubChem | |
Molecular Formula |
Cl3Si | |
Source | PubChem | |
URL | https://pubchem.ncbi.nlm.nih.gov | |
Description | Data deposited in or computed by PubChem | |
DSSTOX Substance ID |
DTXSID4029301, DTXSID40940810 | |
Record name | Trichlorosilane | |
Source | EPA DSSTox | |
URL | https://comptox.epa.gov/dashboard/DTXSID4029301 | |
Description | DSSTox provides a high quality public chemistry resource for supporting improved predictive toxicology. | |
Record name | Trichlorosilyl | |
Source | EPA DSSTox | |
URL | https://comptox.epa.gov/dashboard/DTXSID40940810 | |
Description | DSSTox provides a high quality public chemistry resource for supporting improved predictive toxicology. | |
Molecular Weight |
134.44 g/mol | |
Source | PubChem | |
URL | https://pubchem.ncbi.nlm.nih.gov | |
Description | Data deposited in or computed by PubChem | |
CAS No. |
19165-34-5, 10025-78-2 | |
Record name | Silyl, trichloro- | |
Source | CAS Common Chemistry | |
URL | https://commonchemistry.cas.org/detail?cas_rn=19165-34-5 | |
Description | CAS Common Chemistry is an open community resource for accessing chemical information. Nearly 500,000 chemical substances from CAS REGISTRY cover areas of community interest, including common and frequently regulated chemicals, and those relevant to high school and undergraduate chemistry classes. This chemical information, curated by our expert scientists, is provided in alignment with our mission as a division of the American Chemical Society. | |
Explanation | The data from CAS Common Chemistry is provided under a CC-BY-NC 4.0 license, unless otherwise stated. | |
Record name | Trichlorosilane | |
Source | ChemIDplus | |
URL | https://pubchem.ncbi.nlm.nih.gov/substance/?source=chemidplus&sourceid=0010025782 | |
Description | ChemIDplus is a free, web search system that provides access to the structure and nomenclature authority files used for the identification of chemical substances cited in National Library of Medicine (NLM) databases, including the TOXNET system. | |
Record name | Trichlorosilyl | |
Source | ChemIDplus | |
URL | https://pubchem.ncbi.nlm.nih.gov/substance/?source=chemidplus&sourceid=0019165345 | |
Description | ChemIDplus is a free, web search system that provides access to the structure and nomenclature authority files used for the identification of chemical substances cited in National Library of Medicine (NLM) databases, including the TOXNET system. | |
Record name | Trichlorosilane | |
Source | EPA DSSTox | |
URL | https://comptox.epa.gov/dashboard/DTXSID4029301 | |
Description | DSSTox provides a high quality public chemistry resource for supporting improved predictive toxicology. | |
Record name | Trichlorosilyl | |
Source | EPA DSSTox | |
URL | https://comptox.epa.gov/dashboard/DTXSID40940810 | |
Description | DSSTox provides a high quality public chemistry resource for supporting improved predictive toxicology. | |
Record name | TRICHLOROSILANE | |
Source | FDA Global Substance Registration System (GSRS) | |
URL | https://gsrs.ncats.nih.gov/ginas/app/beta/substances/QZY2645L6V | |
Description | The FDA Global Substance Registration System (GSRS) enables the efficient and accurate exchange of information on what substances are in regulated products. Instead of relying on names, which vary across regulatory domains, countries, and regions, the GSRS knowledge base makes it possible for substances to be defined by standardized, scientific descriptions. | |
Explanation | Unless otherwise noted, the contents of the FDA website (www.fda.gov), both text and graphics, are not copyrighted. They are in the public domain and may be republished, reprinted and otherwise used freely by anyone without the need to obtain permission from FDA. Credit to the U.S. Food and Drug Administration as the source is appreciated but not required. | |
体外研究产品的免责声明和信息
请注意,BenchChem 上展示的所有文章和产品信息仅供信息参考。 BenchChem 上可购买的产品专为体外研究设计,这些研究在生物体外进行。体外研究,源自拉丁语 "in glass",涉及在受控实验室环境中使用细胞或组织进行的实验。重要的是要注意,这些产品没有被归类为药物或药品,他们没有得到 FDA 的批准,用于预防、治疗或治愈任何医疗状况、疾病或疾病。我们必须强调,将这些产品以任何形式引入人类或动物的身体都是法律严格禁止的。遵守这些指南对确保研究和实验的法律和道德标准的符合性至关重要。