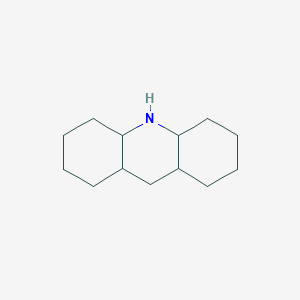
Tetradecahydroacridine
描述
Tetradecahydroacridine is a chemical compound with the molecular formula C₁₃H₂₃N It is a saturated azaheterocyclic tertiary amine, which means it contains a nitrogen atom within a ring structure that is fully saturated with hydrogen atoms
准备方法
Synthetic Routes and Reaction Conditions
The synthesis of tetradecahydroacridine can be achieved through the reductive amination of (R*,R*)-2,2’-methylene-bis-cyclohexanone with methylamine and potassium borohydride. This reaction yields a mixture of (4aα,8aβ,9aα,10aβ)- and (4aα,8aα,9aβ,10aα)-tetradecahydro-10-methylacridine in a ratio of approximately 1.3:1 with an overall yield of 57% . The N-demethylation of these intermediates via the N-nitrosamine pathway leads to the formation of this compound .
Industrial Production Methods
Industrial production methods for this compound are not well-documented in the literature. the synthetic routes mentioned above can be scaled up for industrial applications with appropriate optimization of reaction conditions and purification processes.
化学反应分析
Types of Reactions
Tetradecahydroacridine undergoes various chemical reactions, including:
Oxidation: The compound can be oxidized under specific conditions to form corresponding oxides.
Reduction: It can be reduced to form different derivatives, depending on the reducing agents used.
Substitution: this compound can undergo substitution reactions, particularly at the nitrogen atom.
Common Reagents and Conditions
Oxidation: Common oxidizing agents include potassium permanganate and hydrogen peroxide.
Reduction: Reducing agents such as lithium aluminum hydride and sodium borohydride are commonly used.
Substitution: Reagents like alkyl halides and acyl chlorides are used for substitution reactions.
Major Products Formed
The major products formed from these reactions depend on the specific reagents and conditions used. For example, oxidation may yield oxides, while reduction can produce various reduced derivatives of this compound.
科学研究应用
Biology: Research is ongoing to explore its potential biological activities and interactions with biological molecules.
Medicine: The compound’s derivatives are being investigated for their potential therapeutic applications, particularly in the treatment of neurodegenerative diseases.
Industry: Tetradecahydroacridine and its derivatives may have applications in the development of new materials and chemical processes.
作用机制
The mechanism of action of tetradecahydroacridine involves its interaction with specific molecular targets and pathways. The compound’s effects are mediated through its ability to undergo various chemical reactions, such as oxidation and reduction, which can alter its structure and reactivity. These changes can influence its interactions with biological molecules and cellular pathways, leading to potential therapeutic effects.
相似化合物的比较
Tetradecahydroacridine can be compared with other similar compounds, such as:
Decahydroquinoline: Similar in structure but with fewer hydrogen atoms.
Tetrahydroacridine: Contains fewer hydrogen atoms and different stereochemical properties.
Hexahydroacridine: Another related compound with different hydrogen saturation levels.
生物活性
Tetradecahydroacridine (THA) is a polycyclic compound belonging to the acridine family, characterized by its unique structure and diverse biological activities. This article explores the biological activity of THA, focusing on its pharmacological effects, mechanisms of action, and relevant case studies.
Chemical Structure and Properties
This compound consists of a fused ring system that contributes to its chemical reactivity and biological properties. The compound can be represented as follows:
This structure allows THA to interact with various biological targets, making it a compound of interest in medicinal chemistry.
1. Antimicrobial Activity
THA derivatives have been shown to exhibit significant antimicrobial properties. Studies indicate that these compounds can inhibit the growth of various bacteria and fungi. For instance, research has demonstrated that certain THA derivatives possess potent activity against strains of Staphylococcus aureus and Escherichia coli, indicating their potential as antimicrobial agents .
2. Antitumor Effects
The anticancer potential of this compound has been explored in several studies. It has been found to induce apoptosis in cancer cells through mechanisms involving DNA intercalation and inhibition of topoisomerases. In vitro studies revealed that THA can reduce cell viability in various cancer cell lines, including breast and lung cancer cells .
3. Neuroprotective Properties
Recent research has highlighted the neuroprotective effects of THA, particularly in models of neurodegenerative diseases such as Alzheimer's. THA derivatives have been investigated for their ability to inhibit acetylcholinesterase (AChE), an enzyme associated with cognitive decline in Alzheimer's disease. This inhibition may enhance cholinergic neurotransmission, providing a therapeutic avenue for cognitive enhancement .
The biological activity of this compound is attributed to several mechanisms:
- DNA Binding : THA can intercalate into DNA, disrupting replication and transcription processes.
- Enzyme Inhibition : The compound acts as an inhibitor of various enzymes, including AChE and topoisomerases.
- Reactive Oxygen Species (ROS) Generation : THA can induce oxidative stress in cells, leading to apoptosis in cancerous cells.
Case Study 1: Antimicrobial Efficacy
In a study published in Thieme Connect, researchers synthesized several THA derivatives and tested their antimicrobial activity against common pathogens. The results indicated that certain modifications to the THA structure significantly enhanced its antibacterial properties, suggesting a structure-activity relationship that could guide future drug development .
Case Study 2: Antitumor Activity
Another study focused on the anticancer effects of THA derivatives in vitro. The researchers treated various cancer cell lines with different concentrations of THA and assessed cell viability using MTT assays. The findings revealed that THA significantly reduced cell viability in a dose-dependent manner, prompting further investigation into its potential as an anticancer agent .
Data Table: Summary of Biological Activities
属性
IUPAC Name |
1,2,3,4,4a,5,6,7,8,8a,9,9a,10,10a-tetradecahydroacridine | |
---|---|---|
Source | PubChem | |
URL | https://pubchem.ncbi.nlm.nih.gov | |
Description | Data deposited in or computed by PubChem | |
InChI |
InChI=1S/C13H23N/c1-3-7-12-10(5-1)9-11-6-2-4-8-13(11)14-12/h10-14H,1-9H2 | |
Source | PubChem | |
URL | https://pubchem.ncbi.nlm.nih.gov | |
Description | Data deposited in or computed by PubChem | |
InChI Key |
IXTPCSZJZAKJTO-UHFFFAOYSA-N | |
Source | PubChem | |
URL | https://pubchem.ncbi.nlm.nih.gov | |
Description | Data deposited in or computed by PubChem | |
Canonical SMILES |
C1CCC2C(C1)CC3CCCCC3N2 | |
Source | PubChem | |
URL | https://pubchem.ncbi.nlm.nih.gov | |
Description | Data deposited in or computed by PubChem | |
Molecular Formula |
C13H23N | |
Source | PubChem | |
URL | https://pubchem.ncbi.nlm.nih.gov | |
Description | Data deposited in or computed by PubChem | |
DSSTOX Substance ID |
DTXSID40937301 | |
Record name | Tetradecahydroacridine | |
Source | EPA DSSTox | |
URL | https://comptox.epa.gov/dashboard/DTXSID40937301 | |
Description | DSSTox provides a high quality public chemistry resource for supporting improved predictive toxicology. | |
Molecular Weight |
193.33 g/mol | |
Source | PubChem | |
URL | https://pubchem.ncbi.nlm.nih.gov | |
Description | Data deposited in or computed by PubChem | |
CAS No. |
16726-19-5, 24526-17-8 | |
Record name | 4aalpha,8abeta,9abeta,10aalpha-Tetradecahydroacridine | |
Source | ChemIDplus | |
URL | https://pubchem.ncbi.nlm.nih.gov/substance/?source=chemidplus&sourceid=0016726195 | |
Description | ChemIDplus is a free, web search system that provides access to the structure and nomenclature authority files used for the identification of chemical substances cited in National Library of Medicine (NLM) databases, including the TOXNET system. | |
Record name | 4aalpha,8abeta,9aalpha,10aalpha-Tetradecahydroacridine | |
Source | ChemIDplus | |
URL | https://pubchem.ncbi.nlm.nih.gov/substance/?source=chemidplus&sourceid=0024526178 | |
Description | ChemIDplus is a free, web search system that provides access to the structure and nomenclature authority files used for the identification of chemical substances cited in National Library of Medicine (NLM) databases, including the TOXNET system. | |
Record name | Tetradecahydroacridine | |
Source | EPA DSSTox | |
URL | https://comptox.epa.gov/dashboard/DTXSID40937301 | |
Description | DSSTox provides a high quality public chemistry resource for supporting improved predictive toxicology. | |
Retrosynthesis Analysis
AI-Powered Synthesis Planning: Our tool employs the Template_relevance Pistachio, Template_relevance Bkms_metabolic, Template_relevance Pistachio_ringbreaker, Template_relevance Reaxys, Template_relevance Reaxys_biocatalysis model, leveraging a vast database of chemical reactions to predict feasible synthetic routes.
One-Step Synthesis Focus: Specifically designed for one-step synthesis, it provides concise and direct routes for your target compounds, streamlining the synthesis process.
Accurate Predictions: Utilizing the extensive PISTACHIO, BKMS_METABOLIC, PISTACHIO_RINGBREAKER, REAXYS, REAXYS_BIOCATALYSIS database, our tool offers high-accuracy predictions, reflecting the latest in chemical research and data.
Strategy Settings
Precursor scoring | Relevance Heuristic |
---|---|
Min. plausibility | 0.01 |
Model | Template_relevance |
Template Set | Pistachio/Bkms_metabolic/Pistachio_ringbreaker/Reaxys/Reaxys_biocatalysis |
Top-N result to add to graph | 6 |
Feasible Synthetic Routes
体外研究产品的免责声明和信息
请注意,BenchChem 上展示的所有文章和产品信息仅供信息参考。 BenchChem 上可购买的产品专为体外研究设计,这些研究在生物体外进行。体外研究,源自拉丁语 "in glass",涉及在受控实验室环境中使用细胞或组织进行的实验。重要的是要注意,这些产品没有被归类为药物或药品,他们没有得到 FDA 的批准,用于预防、治疗或治愈任何医疗状况、疾病或疾病。我们必须强调,将这些产品以任何形式引入人类或动物的身体都是法律严格禁止的。遵守这些指南对确保研究和实验的法律和道德标准的符合性至关重要。