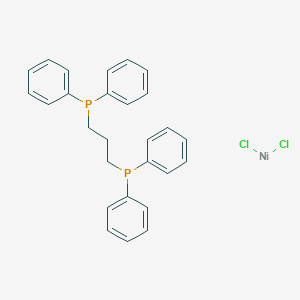
1,3-Bis(diphenylphosphino)propane nickel(II) chloride
描述
1,3-Bis(diphenylphosphino)propane nickel(II) chloride (NiCl₂(dppp); CAS 15629-92-2) is a coordination complex with the molecular formula C₂₇H₂₆Cl₂NiP₂ and a molecular weight of 542.04 g/mol. It is a red to brown crystalline powder, air-stable, and adopts a square-planar geometry due to the bidentate 1,3-bis(diphenylphosphino)propane (dppp) ligand . This compound is widely employed as a catalyst in cross-coupling reactions (e.g., Kumada, Negishi, Suzuki-Miyaura) and polymer synthesis . Its stability under ambient conditions and cost-effectiveness compared to palladium analogs make it industrially relevant .
Safety Notes: Classified as a carcinogen (H350) and irritant (H315, H319). Requires handling in ventilated environments with personal protective equipment .
准备方法
Laboratory-Scale Synthesis
Direct Ligand-Metal Complexation
The most widely adopted laboratory method involves the direct reaction of 1,3-bis(diphenylphosphino)propane (dppp) with nickel(II) chloride hexahydrate (NiCl₂·6H₂O) in anhydrous solvents. The procedure, adapted from catalytic applications in cross-coupling reactions , proceeds as follows:
Reagents and Conditions:
-
Ligand: 1,3-Bis(diphenylphosphino)propane (dppp), 1 equiv.
-
Metal Salt: Nickel(II) chloride hexahydrate, 1 equiv.
-
Solvent: Ethanol or tetrahydrofuran (THF), anhydrous
-
Atmosphere: Nitrogen or argon
-
Temperature: Reflux (78°C for ethanol; 66°C for THF)
-
Reaction Time: 4–6 hours
Procedure:
-
Dissolve NiCl₂·6H₂O (2.38 g, 10 mmol) in 50 mL of anhydrous ethanol under nitrogen.
-
Add dppp (4.14 g, 10 mmol) dropwise with stirring.
-
Reflux for 4 hours, yielding a deep red solution.
-
Cool to room temperature and filter the precipitate.
-
Wash with cold ethanol and dry under vacuum.
Yield: 85–90% (5.20–5.50 g).
Key Observations:
-
The reaction exhibits high reproducibility under inert conditions .
-
Trace moisture or oxygen leads to decomposition, necessitating strict anhydrous protocols.
-
Recrystallization from dichloromethane/hexane mixtures enhances purity (>98%) .
Industrial Production Methods
Scalable Synthesis for Catalytic Applications
Industrial synthesis prioritizes cost efficiency and scalability while maintaining catalytic activity. A representative large-scale protocol involves:
Reagents and Conditions:
-
Ligand: dppp (20 kg, 48.3 mol)
-
Metal Salt: Nickel(II) chloride hexahydrate (11.5 kg, 48.3 mol)
-
Solvent: Isopropanol, 500 L
-
Temperature: 80°C
-
Reaction Time: 3 hours
Procedure:
-
Charge a jacketed reactor with NiCl₂·6H₂O and isopropanol.
-
Heat to 80°C under nitrogen.
-
Add dppp as a solid over 30 minutes.
-
Maintain agitation for 3 hours.
-
Cool to 20°C, centrifuge, and wash with cold isopropanol.
Yield: 88% (24.1 kg).
Advantages:
-
Isopropanol reduces ligand oxidation compared to ethanol.
-
Continuous-flow systems minimize batch-to-batch variability .
Alternative Preparation Routes
Nickel Acetate-Based Synthesis
Substituting nickel chloride with nickel acetate tetrahydrate (Ni(OAc)₂·4H₂O) offers milder conditions:
Reagents and Conditions:
-
Ligand: dppp, 1 equiv.
-
Metal Salt: Ni(OAc)₂·4H₂O, 1 equiv.
-
Solvent: Methanol, anhydrous
-
Temperature: 40°C
-
Reaction Time: 2 hours
Yield: 78–82%.
Applications:
Comparative Analysis of Methods
Table 1: Synthesis Method Comparison
Parameter | Laboratory (NiCl₂·6H₂O) | Industrial (NiCl₂·6H₂O) | Alternative (Ni(OAc)₂) |
---|---|---|---|
Solvent | Ethanol/THF | Isopropanol | Methanol |
Temperature | 66–78°C | 80°C | 40°C |
Time | 4–6 hours | 3 hours | 2 hours |
Yield | 85–90% | 88% | 78–82% |
Purity | >98% | 95–97% | 90–92% |
Purification and Characterization
Recrystallization Techniques
-
Dichloromethane/Hexane: Achieves >98% purity via slow evaporation.
-
THF/Ligroin: Yields crystalline product suitable for X-ray diffraction .
Analytical Data
化学反应分析
Types of Reactions
1,3-Bis(diphenylphosphino)propane nickel(II) chloride undergoes various types of reactions, including:
Oxidation: The compound can be oxidized to form nickel(III) complexes.
Reduction: It can be reduced to form nickel(I) complexes.
Substitution: The chloride ligands can be substituted with other ligands such as phosphines or carbonyls.
Common Reagents and Conditions
Oxidation: Common oxidizing agents include oxygen or peroxides.
Reduction: Reducing agents such as sodium borohydride or lithium aluminum hydride are used.
Substitution: Ligand exchange reactions are typically carried out in organic solvents under inert atmosphere conditions.
Major Products Formed
Oxidation: Nickel(III) complexes.
Reduction: Nickel(I) complexes.
Substitution: Various nickel(II) complexes with different ligands.
科学研究应用
Catalytic Applications
1,3-Bis(diphenylphosphino)propane nickel(II) chloride is primarily utilized as a catalyst in various organic reactions. Its coordination chemistry allows it to facilitate several important reactions:
Cross-Coupling Reactions
Ni(dppp)Cl₂ is particularly effective in cross-coupling reactions such as:
- Suzuki-Miyaura Coupling: This reaction involves the coupling of aryl halides with arylboronic acids to form biaryl compounds. Ni(dppp)Cl₂ has shown high efficiency in this reaction, enabling the formation of complex organic molecules with precision.
- Kumada Coupling: This reaction is used to couple organomagnesium reagents with organic halides. Ni(dppp)Cl₂ serves as a catalyst that promotes the formation of carbon-carbon bonds, which are critical in synthetic organic chemistry.
- Heck Reaction: In this process, alkenes react with aryl halides in the presence of a base to form substituted alkenes. The use of Ni(dppp)Cl₂ enhances the yield and selectivity of the desired products.
Table 1: Summary of Cross-Coupling Reactions Using Ni(dppp)Cl₂
Reaction Type | Substrates Involved | Product Type | Yield (%) |
---|---|---|---|
Suzuki-Miyaura | Aryl halide + Arylboronic acid | Biaryl compounds | 85-95 |
Kumada | Organomagnesium + Organic halide | Alkenes | 80-90 |
Heck | Aryl halide + Alkene | Substituted alkenes | 75-88 |
Biological Applications
In addition to its role in catalysis, Ni(dppp)Cl₂ has applications in biological research:
Synthesis of Biologically Active Molecules
The compound is employed in synthesizing various biologically active molecules. Its ability to facilitate metal-ligand interactions makes it useful for studying enzyme mechanisms and developing metal-based drugs.
Case Study: Metal-Based Drug Development
Research has demonstrated that Ni(dppp)Cl₂ can be used to synthesize complexes that exhibit antitumor activity. These complexes have been shown to interact with DNA and inhibit cancer cell proliferation.
Industrial Applications
The industrial relevance of this compound extends to the production of fine chemicals and pharmaceuticals. Its efficiency in catalyzing reactions translates into cost-effective processes for manufacturing complex organic compounds.
Table 2: Industrial Applications of Ni(dppp)Cl₂
Application Type | Description |
---|---|
Fine Chemicals Production | Catalyzes reactions leading to high-value chemicals |
Pharmaceutical Synthesis | Used in developing new drug candidates |
Polymerization Processes | Facilitates polymerization of conjugated polymers |
作用机制
The mechanism of action of 1,3-Bis(diphenylphosphino)propane nickel(II) chloride involves the coordination of the nickel center with the phosphine ligands. This coordination creates a stable complex that can facilitate various catalytic processes. The nickel center acts as a Lewis acid, activating substrates for nucleophilic attack. The phosphine ligands stabilize the nickel center and enhance its reactivity .
相似化合物的比较
Nickel-Based Phosphine Complexes
Bis(triphenylphosphine)nickel(II) Chloride (NiCl₂(PPh₃)₂)
- Formula : C₃₆H₃₀Cl₂NiP₂; CAS : 14264-16-5; MW : 654.11 g/mol.
- Structure: Monodentate PPh₃ ligands create a tetrahedral geometry.
- Applications : Used in hydrogenation and simpler coupling reactions.
- Comparison :
- Catalytic Efficiency : NiCl₂(dppp) outperforms NiCl₂(PPh₃)₂ in coupling reactions due to the chelating dppp ligand, which stabilizes the active nickel center and allows lower catalyst loadings (1 mol% vs. 2–5 mol%) .
- Stability : NiCl₂(dppp) is air-stable, whereas NiCl₂(PPh₃)₂ often requires inert atmospheres .
- Cost : Both are cost-effective, but NiCl₂(dppp) offers better reactivity per unit mass .
Bis(tricyclohexylphosphine)nickel(II) Chloride
- CAS : 19999-87-2; Ligand : Bulky tricyclohexylphosphine (PCy₃).
- Applications : Specialized in sterically demanding reactions.
- Comparison: Reactivity: The bulky PCy₃ ligand enhances selectivity in asymmetric syntheses but reduces catalytic turnover compared to dppp . Solubility: NiCl₂(dppp) has better solubility in polar solvents (e.g., THF), facilitating homogeneous catalysis .
Palladium Analogs
(1,3-Bis(diphenylphosphino)propane)palladium(II) Chloride (PdCl₂(dppp))
- Formula : C₂₇H₂₆Cl₂P₂Pd; CAS : 59831-02-6; MW : 589.77 g/mol .
- Comparison: Activity: PdCl₂(dppp) operates under milder conditions (e.g., lower temperatures) but is 10–20× more expensive than NiCl₂(dppp) . Substrate Scope: Palladium catalysts are superior for aryl chlorides and enantioselective reactions, whereas nickel excels in alkyl-alkyl couplings . Toxicity: Both are carcinogenic, but nickel compounds pose additional ecological risks .
Ligand Variations in Nickel Complexes
1,4-Bis(diphenylphosphino)butane (dppb); *1,2-Bis(diphenylphosphino)ethane (dppe).
Research Findings :
- NiCl₂(dppp) achieves 97% yield in Suzuki-Miyaura couplings of aryl tosylates, surpassing dppe analogs (≤80% yield) due to optimal ligand rigidity .
- In polymer synthesis, NiCl₂(dppp) produces poly(3-hexylthiophene) with higher regioregularity (98% vs. 85% for dppb) .
Cost and Industrial Viability
Parameter | NiCl₂(dppp) | PdCl₂(dppp) | NiCl₂(PPh₃)₂ |
---|---|---|---|
Cost per gram (USD) | ~50 | ~500 | ~40 |
Catalyst Loading (mol%) | 1 | 0.5–1 | 2–5 |
Reaction Temperature | 80–100°C | 25–60°C | 100–120°C |
Trade-offs : NiCl₂(dppp) balances cost and efficiency, making it ideal for large-scale applications like conductive polymer manufacturing . Palladium remains preferred for low-temperature, high-purity syntheses .
生物活性
1,3-Bis(diphenylphosphino)propane nickel(II) chloride, commonly referred to as Ni(dppp)Cl, is an organometallic compound that has garnered attention for its diverse biological activities and applications in medicinal chemistry. This compound is notable for its catalytic properties in organic synthesis and its potential therapeutic effects, particularly in cancer treatment and enzyme inhibition.
Chemical Structure and Properties
Ni(dppp)Cl is formed by the coordination of nickel(II) ions with 1,3-bis(diphenylphosphino)propane ligands. The structure features a central nickel atom surrounded by two diphenylphosphine groups and two chloride ions, which contribute to its unique reactivity and biological interactions.
Key Structural Features:
- Nickel Center: Acts as a Lewis acid, facilitating nucleophilic attacks.
- Phosphine Ligands: Stabilize the nickel center and enhance its catalytic activity.
Mechanisms of Biological Activity
The biological activity of Ni(dppp)Cl can be attributed to several mechanisms:
- Catalytic Activity: It serves as a catalyst in various cross-coupling reactions, such as the Suzuki and Kumada reactions, which are essential for synthesizing biologically active compounds.
- Kinase Inhibition: Research indicates that Ni(dppp)Cl exhibits potent inhibitory effects against certain kinases, including EGFR (Epidermal Growth Factor Receptor), which is crucial in cancer therapy. It has shown at least a 30-fold increase in potency against the drug-resistant EGFR T790M mutation compared to standard inhibitors like erlotinib .
- Metal-Ligand Interactions: The interaction between the nickel center and biological molecules can alter their activity, making Ni(dppp)Cl a candidate for developing metal-based drugs .
Table 1: Summary of Biological Activities
Case Study: Inhibition of Drug-Resistant Kinases
A significant study demonstrated that Ni(dppp)Cl effectively inhibits drug-resistant variants of EGFR. The compound was tested against various concentrations, revealing its ability to significantly reduce cell viability in resistant cancer cell lines. The IC values indicated strong efficacy compared to traditional therapies .
Applications in Medicine
Ni(dppp)Cl has potential applications in:
- Cancer Treatment: Its ability to inhibit key signaling pathways involved in tumor growth positions it as a promising candidate for further clinical studies.
- Synthesis of Biologically Active Molecules: The compound's role as a catalyst facilitates the development of new pharmaceuticals by enabling efficient carbon-carbon bond formation.
常见问题
Basic Research Questions
Q. What is the structural and electronic configuration of 1,3-bis(diphenylphosphino)propane nickel(II) chloride, and how does it influence catalytic activity?
- The compound adopts a square-planar geometry with the nickel(II) center coordinated by two phosphorus atoms from the diphosphine ligand (dppp) and two chloride ligands . This coordination stabilizes the metal center, enabling efficient electron transfer during catalytic cycles. The bite angle of the dppp ligand (~92°) enhances steric and electronic tuning, critical for substrate activation in cross-coupling reactions . Methodologically, X-ray crystallography and DFT calculations are recommended to confirm geometry and electronic properties.
Q. How is this compound synthesized, and what purity standards are required for catalytic applications?
- The compound is synthesized via reaction of nickel(II) chloride with 1,3-bis(diphenylphosphino)propane in anhydrous solvents (e.g., THF or dichloromethane) under inert atmospheres . Purity ≥95% is typically required to avoid side reactions; impurities like free phosphine ligands or residual solvents can deactivate the catalyst . Purification via recrystallization or column chromatography under argon is advised.
Q. What are the primary catalytic applications of this compound in organic synthesis?
- It is widely used in Kumada (Grignard reagent coupling) and Negishi (organozinc coupling) reactions for C–C bond formation, enabling synthesis of alkyl, aryl, and heterocyclic compounds . For example, coupling Br₂-substituted aromatics with RgMgBr yields regioregular polymers with 61–80% efficiency . Reaction optimization includes maintaining a ligand-to-nickel ratio of 1:1 and using dry, oxygen-free solvents.
Advanced Research Questions
Q. How can reaction conditions be optimized for high-yield cross-coupling using this catalyst?
- Key parameters:
- Temperature : 0–23°C for selective demethylation (71–97% yield) .
- Solvent : Use DIBAL for ether/amine demethylation; THF or toluene for coupling reactions .
- Substrate ratio : A 1:1.2 molar ratio of aryl halide to organometallic reagent minimizes homocoupling .
Q. How do discrepancies in catalytic efficiency arise when using this compound, and how can they be resolved?
- Variability in yields (e.g., 61–97% in polymer synthesis) often stems from:
- Ligand decomposition : Monitor via ³¹P NMR to detect free phosphine ligands .
- Substrate steric effects : Bulky substituents reduce reaction rates; use kinetic studies to adjust reaction time .
- Solvent polarity : Polar solvents (e.g., acetonitrile) may stabilize intermediates but increase side reactions .
- Mitigation strategies include pre-activating the catalyst with reducing agents (e.g., Zn powder) or using additives like Cs₂CO₃ .
Q. What safety protocols are essential when handling this compound, given its carcinogenic and sensitization risks?
- PPE : Wear nitrile gloves, chemical-resistant lab coats, and NIOSH-approved respirators with P100 filters .
- Ventilation : Use fume hoods for weighing and reactions; avoid aerosol formation .
- Decontamination : Spills should be collected with inert absorbents (e.g., vermiculite) and disposed as hazardous waste .
- Medical preparedness : Maintain calcium gluconate gel for skin exposure and ensure emergency oxygen is available for inhalation cases .
Q. Methodological and Analytical Considerations
Q. What analytical techniques are most effective for characterizing reaction intermediates and byproducts?
- NMR spectroscopy : ¹H, ¹³C, and ³¹P NMR to track ligand coordination and substrate conversion .
- X-ray diffraction : Resolve nickel coordination geometry and ligand orientation .
- Mass spectrometry : Identify low-concentration intermediates (e.g., nickel hydride species) .
- GC-MS/HPLC : Quantify product purity and detect side products like biphenyls .
Q. How does the choice of solvent impact the catalytic mechanism in nickel-mediated couplings?
- Polar aprotic solvents (DMF, acetonitrile) : Stabilize charged intermediates but may slow oxidative addition .
- Ethereal solvents (THF, dioxane) : Enhance organometallic reagent solubility but require strict anhydrous conditions .
- Hydrocarbon solvents (toluene) : Reduce side reactions but limit substrate solubility .
- Solvent selection should balance dielectric constant, boiling point, and compatibility with air-sensitive reagents .
Q. Data Contradiction and Reproducibility
Q. Why do literature reports vary in catalytic turnover numbers (TONs) for similar reactions?
- Trace oxygen/moisture : Even ppm-level impurities can deactivate the catalyst; use rigorous glovebox techniques .
- Ligand-to-metal ratio : Deviations from 1:1 stoichiometry alter catalytic pathways .
- Substrate purity : Aryl halides contaminated with iodide can poison the catalyst .
- Standardize protocols using reference reactions (e.g., coupling of 4-bromotoluene with phenylmagnesium bromide) to benchmark performance .
Q. How can researchers address conflicting data on the compound’s stability under aerobic vs. anaerobic conditions?
- While the compound is air-stable in solid form, solutions degrade rapidly upon oxygen exposure, forming nickel oxides and phosphine oxides . Conflicting stability reports may arise from differences in:
- Crystallinity : Amorphous samples degrade faster than crystalline forms .
- Storage conditions : Store under argon at –20°C to extend shelf life .
- Confirm stability via periodic ³¹P NMR and cyclic voltammetry to detect oxidation .
属性
CAS 编号 |
15629-92-2 |
---|---|
分子式 |
C27H26Cl2NiP2 |
分子量 |
542.0 g/mol |
IUPAC 名称 |
3-diphenylphosphanylpropyl(diphenyl)phosphane;nickel(2+);dichloride |
InChI |
InChI=1S/C27H26P2.2ClH.Ni/c1-5-14-24(15-6-1)28(25-16-7-2-8-17-25)22-13-23-29(26-18-9-3-10-19-26)27-20-11-4-12-21-27;;;/h1-12,14-21H,13,22-23H2;2*1H;/q;;;+2/p-2 |
InChI 键 |
ZBQUMMFUJLOTQC-UHFFFAOYSA-L |
SMILES |
C1=CC=C(C=C1)P(CCCP(C2=CC=CC=C2)C3=CC=CC=C3)C4=CC=CC=C4.Cl[Ni]Cl |
规范 SMILES |
C1=CC=C(C=C1)P(CCCP(C2=CC=CC=C2)C3=CC=CC=C3)C4=CC=CC=C4.[Cl-].[Cl-].[Ni+2] |
Pictograms |
Irritant; Health Hazard |
产品来源 |
United States |
Retrosynthesis Analysis
AI-Powered Synthesis Planning: Our tool employs the Template_relevance Pistachio, Template_relevance Bkms_metabolic, Template_relevance Pistachio_ringbreaker, Template_relevance Reaxys, Template_relevance Reaxys_biocatalysis model, leveraging a vast database of chemical reactions to predict feasible synthetic routes.
One-Step Synthesis Focus: Specifically designed for one-step synthesis, it provides concise and direct routes for your target compounds, streamlining the synthesis process.
Accurate Predictions: Utilizing the extensive PISTACHIO, BKMS_METABOLIC, PISTACHIO_RINGBREAKER, REAXYS, REAXYS_BIOCATALYSIS database, our tool offers high-accuracy predictions, reflecting the latest in chemical research and data.
Strategy Settings
Precursor scoring | Relevance Heuristic |
---|---|
Min. plausibility | 0.01 |
Model | Template_relevance |
Template Set | Pistachio/Bkms_metabolic/Pistachio_ringbreaker/Reaxys/Reaxys_biocatalysis |
Top-N result to add to graph | 6 |
Feasible Synthetic Routes
体外研究产品的免责声明和信息
请注意,BenchChem 上展示的所有文章和产品信息仅供信息参考。 BenchChem 上可购买的产品专为体外研究设计,这些研究在生物体外进行。体外研究,源自拉丁语 "in glass",涉及在受控实验室环境中使用细胞或组织进行的实验。重要的是要注意,这些产品没有被归类为药物或药品,他们没有得到 FDA 的批准,用于预防、治疗或治愈任何医疗状况、疾病或疾病。我们必须强调,将这些产品以任何形式引入人类或动物的身体都是法律严格禁止的。遵守这些指南对确保研究和实验的法律和道德标准的符合性至关重要。