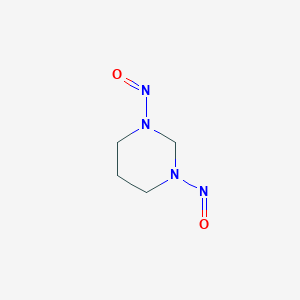
Di(N-nitroso)-perhydropyrimidine
描述
Di(N-nitroso)-perhydropyrimidine (DNPP) is a synthetic N-nitroso compound characterized by a perhydropyrimidine backbone with two nitroso (N–N=O) functional groups. It has been studied extensively for its carcinogenic properties in animal models. In a seminal study by Habs et al. (1983), DNPP was administered intraperitoneally to Sprague-Dawley rats at doses of 15 mg/kg and 30 mg/kg weekly for life. The compound significantly reduced lifespan and induced esophageal tumors (27–33%) and liver tumors (4–8%) in both dose groups . These findings classify DNPP as a potent carcinogen, with a unique propensity for esophageal carcinogenesis compared to other N-nitroso compounds.
准备方法
Synthetic Routes and Reaction Conditions
The synthesis of Di(N-nitroso)-perhydropyrimidine typically involves the nitrosation of secondary amines. One common method is the reaction of perhydropyrimidine with nitrosating agents such as sodium nitrite (NaNO2) in the presence of an acid like hydrochloric acid (HCl). The reaction is usually carried out under mild conditions, often at room temperature, to ensure high yields and purity of the product .
Industrial Production Methods
Industrial production of this compound follows similar synthetic routes but on a larger scale. The process involves the careful control of reaction parameters, such as temperature, pressure, and concentration of reactants, to optimize yield and minimize by-products. The use of continuous flow reactors and automated systems can enhance the efficiency and scalability of the production process .
化学反应分析
Types of Reactions
Di(N-nitroso)-perhydropyrimidine undergoes various chemical reactions, including:
Oxidation: The compound can be oxidized to form nitro derivatives.
Reduction: Reduction reactions can convert the nitroso groups to amines.
Substitution: The nitroso groups can be substituted by other nucleophiles, leading to the formation of different derivatives
Common Reagents and Conditions
Common reagents used in these reactions include oxidizing agents like potassium permanganate (KMnO4) for oxidation, reducing agents such as sodium borohydride (NaBH4) for reduction, and nucleophiles like amines or thiols for substitution reactions. The reactions are typically carried out under controlled conditions to ensure selectivity and high yields .
Major Products
The major products formed from these reactions depend on the specific reaction conditions and reagents used. For example, oxidation of this compound can yield nitro derivatives, while reduction can produce amine derivatives. Substitution reactions can lead to a variety of functionalized compounds .
科学研究应用
Carcinogenicity Studies
Research has demonstrated that Di(N-nitroso)-perhydropyrimidine exhibits significant carcinogenic properties. In a study involving male Sprague-Dawley rats, doses of 30 mg/kg and 15 mg/kg were administered intraperitoneally. The results indicated a notable increase in tumor incidence, underscoring the compound's potential as a carcinogen .
Photohydrolytic Detection
Recent advancements in analytical techniques have enabled the photohydrolytic detection of N-nitroso compounds, including this compound. High-performance liquid chromatography (HPLC) methods have been developed to quantify these compounds in various matrices, facilitating further research into their environmental and biological impacts .
Therapeutic Potential
While primarily studied for its toxicological effects, there is emerging interest in exploring this compound's potential therapeutic applications. Research suggests that modifications to its structure could yield derivatives with beneficial pharmacological properties, particularly in targeting specific diseases or conditions .
Case Study 1: Carcinogenic Risk Assessment
A comprehensive risk assessment was conducted to evaluate the carcinogenic potential of this compound. The study utilized dose-response modeling to predict human health risks associated with exposure to this compound. Findings indicated a significant correlation between exposure levels and increased cancer risk, highlighting the importance of regulatory measures .
Case Study 2: Environmental Impact
Investigations into the environmental persistence of this compound revealed its potential to contaminate water sources. The compound's stability under various environmental conditions raises concerns about long-term exposure risks to human populations and wildlife .
作用机制
The mechanism of action of Di(N-nitroso)-perhydropyrimidine involves its interaction with molecular targets through its nitroso groups. These interactions can lead to the formation of covalent bonds with nucleophilic sites on proteins, DNA, and other biomolecules. The compound’s reactivity is influenced by its electronic structure and the presence of the nitroso groups, which can participate in various chemical reactions, including redox processes and nucleophilic substitutions .
相似化合物的比较
Comparison with Similar N-Nitroso Compounds
N-nitroso compounds are a broad class of carcinogens divided into nitrosamines (e.g., NDMA, NPYR) and nitrosamides (e.g., N-nitrosoureas). Below, DNPP is compared to structurally and functionally related compounds based on carcinogenicity, exposure routes, and biological mechanisms.
Carcinogenic Profiles
Exposure Pathways and Formation
- Dietary N-Nitroso Compounds (e.g., NDMA, NPIP) : Formed in cured meats (bacon, sausage) via reactions between nitrites and amines . NDMA intake in humans averages 0.3–1.0 µg/day .
- Endogenous N-Nitroso Compounds (e.g., NPRO): Synthesized in the stomach from dietary nitrate and amines, modulated by pH and inhibitors like vitamin C .
Mechanistic Differences
- NDMA/NPIP : Require cytochrome P450-mediated activation to form DNA-reactive methyldiazonium ions, primarily targeting hepatic tissues .
- NPRO: Non-carcinogenic in humans but serves as a biomarker for endogenous nitrosation; linked to gastric cancer risk in high-nitrate diets .
Human Relevance
常见问题
Basic Research Questions
Q. What are the primary mechanisms of Di(N-nitroso)-perhydropyrimidine formation in pharmaceutical synthesis?
this compound forms via nitrosation reactions between secondary amines (e.g., perhydropyrimidine derivatives) and nitrosating agents like nitrous acid or nitrites. Reaction kinetics depend on pH, temperature, and catalytic factors (e.g., thiocyanate ions). Analytical methods such as HPLC-HRMS and nuclear magnetic resonance (NMR) are critical for tracking intermediates and confirming structures .
Q. Which analytical techniques are most reliable for detecting N-nitroso impurities like this compound in drug substances?
High-performance liquid chromatography coupled with mass spectrometry (HPLC-MS) is the gold standard, offering sensitivity down to 0.1 ppm. Thermogravimetric analysis (TGA) under nitrogen flow (25–850°C, 10°C/min heating rate) can assess thermal stability and decomposition products. Regulatory guidelines (e.g., EMA, FDA) recommend these methods for impurity profiling .
Q. How do dietary and environmental exposures to N-nitroso compounds inform toxicity studies of this compound?
Epidemiological studies use food frequency questionnaires (FFQs) and 7-day food records (7DFR) to correlate N-nitroso intake (e.g., cured meats, fish) with cancer risk. However, FFQs may overestimate intake compared to 7DFR, requiring energy-adjusted correlations (Spearman ρ: 0.004–0.48) and tertile-based analysis for validation .
Advanced Research Questions
Q. How can researchers resolve contradictions in carcinogenicity data for this compound across experimental models?
Discrepancies arise from variations in metabolic activation (e.g., cytochrome P450 vs. bacterial nitroreductases) and DNA adduct profiles (e.g., N7-guanine vs. O6-alkylguanine). Mechanistic studies using isotopic labeling and transgenic rodent models (e.g., Mgmt knockout mice) can clarify tissue-specific mutagenicity .
Q. What experimental strategies mitigate N-nitroso compound formation during drug synthesis?
Incorporating nitrosation inhibitors (e.g., ascorbic acid at 1:1 molar ratio with nitrite) blocks secondary amine nitrosation. Process optimization (e.g., low-temperature reactions, inert atmospheres) and real-time monitoring via in situ FTIR spectroscopy reduce impurity formation .
Q. How can surrogate compounds improve risk assessment for this compound when carcinogenicity data are limited?
Surrogates with structural and reactivity similarities (e.g., N-nitroso-duloxetine) enable read-across analysis. Regulatory agencies accept surrogates if they share metabolic pathways (e.g., α-hydroxylation to diazonium ions) and demonstrate comparable DNA adduct profiles in vitro .
Q. What methodologies validate the endogenous formation of this compound in biological systems?
Stable isotope tracing (e.g., ¹⁵N-labeled nitrites) combined with LC-MS/MS quantifies endogenous nitrosation. In vivo models with germ-free rodents or antibiotic-treated subjects distinguish microbial vs. mammalian metabolic contributions .
Q. Methodological Considerations
Q. Designing dose-response studies for this compound: How are "no-effect levels" determined?
Linearized multistage models and benchmark dose (BMD) approaches are used, with thresholds set at <1% excess cancer risk over background. Subchronic rodent studies (90-day exposure) identify provisional tolerable daily intake (TDI) values, adjusted for interspecies differences using allometric scaling .
Q. What computational tools predict the reactivity of this compound in complex matrices?
Density functional theory (DFT) calculates activation energies for nitrosamine formation, while QSAR models correlate structural alerts (e.g., steric hindrance at the nitroso group) with mutagenic potency. Databases like Lhasa Limited’s Derek Nexus prioritize high-risk compounds for testing .
Q. How are conflicting biomarker data (e.g., urinary N-nitroso metabolites) reconciled in exposure studies?
Multivariate regression adjusts for confounding factors (e.g., smoking, pH-dependent nitrosation in urine). Paired analysis of pre- and post-exposure samples and use of stable isotopes (e.g., ¹⁵N-nitrosoproline) improve specificity .
属性
IUPAC Name |
1,3-dinitroso-1,3-diazinane | |
---|---|---|
Source | PubChem | |
URL | https://pubchem.ncbi.nlm.nih.gov | |
Description | Data deposited in or computed by PubChem | |
InChI |
InChI=1S/C4H8N4O2/c9-5-7-2-1-3-8(4-7)6-10/h1-4H2 | |
Source | PubChem | |
URL | https://pubchem.ncbi.nlm.nih.gov | |
Description | Data deposited in or computed by PubChem | |
InChI Key |
JSPJZBZNGPDBLH-UHFFFAOYSA-N | |
Source | PubChem | |
URL | https://pubchem.ncbi.nlm.nih.gov | |
Description | Data deposited in or computed by PubChem | |
Canonical SMILES |
C1CN(CN(C1)N=O)N=O | |
Source | PubChem | |
URL | https://pubchem.ncbi.nlm.nih.gov | |
Description | Data deposited in or computed by PubChem | |
Molecular Formula |
C4H8N4O2 | |
Source | PubChem | |
URL | https://pubchem.ncbi.nlm.nih.gov | |
Description | Data deposited in or computed by PubChem | |
DSSTOX Substance ID |
DTXSID9021009 | |
Record name | di(N-Nitroso)-perhydropyrimidine | |
Source | EPA DSSTox | |
URL | https://comptox.epa.gov/dashboard/DTXSID9021009 | |
Description | DSSTox provides a high quality public chemistry resource for supporting improved predictive toxicology. | |
Molecular Weight |
144.13 g/mol | |
Source | PubChem | |
URL | https://pubchem.ncbi.nlm.nih.gov | |
Description | Data deposited in or computed by PubChem | |
CAS No. |
15973-99-6 | |
Record name | Di(N-nitroso)-perhydropyrimidine | |
Source | ChemIDplus | |
URL | https://pubchem.ncbi.nlm.nih.gov/substance/?source=chemidplus&sourceid=0015973996 | |
Description | ChemIDplus is a free, web search system that provides access to the structure and nomenclature authority files used for the identification of chemical substances cited in National Library of Medicine (NLM) databases, including the TOXNET system. | |
Record name | di(N-Nitroso)-perhydropyrimidine | |
Source | EPA DSSTox | |
URL | https://comptox.epa.gov/dashboard/DTXSID9021009 | |
Description | DSSTox provides a high quality public chemistry resource for supporting improved predictive toxicology. | |
Retrosynthesis Analysis
AI-Powered Synthesis Planning: Our tool employs the Template_relevance Pistachio, Template_relevance Bkms_metabolic, Template_relevance Pistachio_ringbreaker, Template_relevance Reaxys, Template_relevance Reaxys_biocatalysis model, leveraging a vast database of chemical reactions to predict feasible synthetic routes.
One-Step Synthesis Focus: Specifically designed for one-step synthesis, it provides concise and direct routes for your target compounds, streamlining the synthesis process.
Accurate Predictions: Utilizing the extensive PISTACHIO, BKMS_METABOLIC, PISTACHIO_RINGBREAKER, REAXYS, REAXYS_BIOCATALYSIS database, our tool offers high-accuracy predictions, reflecting the latest in chemical research and data.
Strategy Settings
Precursor scoring | Relevance Heuristic |
---|---|
Min. plausibility | 0.01 |
Model | Template_relevance |
Template Set | Pistachio/Bkms_metabolic/Pistachio_ringbreaker/Reaxys/Reaxys_biocatalysis |
Top-N result to add to graph | 6 |
Feasible Synthetic Routes
体外研究产品的免责声明和信息
请注意,BenchChem 上展示的所有文章和产品信息仅供信息参考。 BenchChem 上可购买的产品专为体外研究设计,这些研究在生物体外进行。体外研究,源自拉丁语 "in glass",涉及在受控实验室环境中使用细胞或组织进行的实验。重要的是要注意,这些产品没有被归类为药物或药品,他们没有得到 FDA 的批准,用于预防、治疗或治愈任何医疗状况、疾病或疾病。我们必须强调,将这些产品以任何形式引入人类或动物的身体都是法律严格禁止的。遵守这些指南对确保研究和实验的法律和道德标准的符合性至关重要。