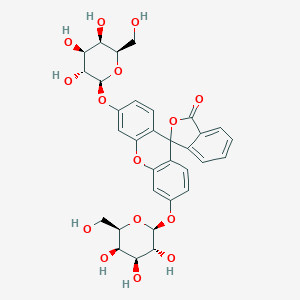
荧光素-二半乳糖苷
描述
荧光素二-β-D-半乳糖苷是一种荧光底物,广泛应用于生化测定。 它对β-半乳糖苷酶特别敏感,这种酶水解该化合物产生高度荧光的产物荧光素 。 这种特性使其成为各种科学研究应用(包括细胞成像和流式细胞术)中宝贵的工具 .
科学研究应用
Key Applications
-
Detection of β-Galactosidase Activity
- FDG serves as an ultra-sensitive substrate for detecting β-galactosidase, particularly in E. coli lacZ gene expression studies.
- It can be utilized in both in vivo and in vitro assays, allowing researchers to quantify enzymatic activity with high precision.
-
Flow Cytometry
- FDG is widely used in flow cytometry for quantifying β-galactosidase activity in gram-negative bacteria and mammalian cells. Its ability to penetrate viable cells enables real-time monitoring of enzyme activity .
- A comparative study indicated that while FDG effectively enters viable cells, its derivative C12-FDG showed limited penetration, highlighting the advantages of FDG in cellular assays .
- Cell Senescence Studies
- Lysosomal Storage Disease Research
- Gene Expression Analysis
Comparative Data Table
Application | Description | Advantages |
---|---|---|
Detection of β-Galactosidase | Sensitive substrate for lacZ gene expression | High sensitivity; suitable for live cell assays |
Flow Cytometry | Quantifies enzyme activity in viable cells | Real-time monitoring; versatile use |
Cell Senescence Studies | Measures senescence-associated β-galactosidase activity | Insights into aging processes |
Lysosomal Storage Disease Research | Investigates diseases linked to deficient lysosomal β-galactosidase activity | Potential for therapeutic insights |
Gene Expression Analysis | Detects β-galactosidase fusion proteins | Useful across various cell types |
Case Studies
-
Flow Cytometric Detection
A study demonstrated the efficiency of FDG in detecting β-galactosidase activity using flow cytometry across different cell types, including mammalian and bacterial cells. The results indicated that FDG provided a fluorescence signal directly proportional to enzymatic activity, facilitating accurate quantification . -
Senescence-Associated Activity Measurement
Researchers employed a fluorimetric method using FDG to quantify β-galactosidase activity associated with cellular senescence in human foreskin fibroblast (Hs68) cells. The findings underscored the utility of FDG in aging research, opening avenues for further exploration into cellular lifespan and health . -
Lysosomal Enzyme Activity Studies
The application of FDG derivatives was explored in the context of lysosomal storage diseases. By utilizing fluorescein-labeled substrates, researchers were able to visualize and quantify enzyme deficiencies linked to specific genetic disorders, providing critical insights into disease mechanisms and potential treatments .
作用机制
荧光素二-β-D-半乳糖苷的作用机制涉及其被β-半乳糖苷酶水解。 该酶裂解糖苷键,释放荧光素单半乳糖苷,随后释放荧光素 。 所得荧光素的荧光强度与酶活性成正比,允许对β-半乳糖苷酶活性进行定量分析 .
生化分析
Biochemical Properties
Fluorescein-digalactoside interacts with various enzymes, proteins, and other biomolecules. The primary enzyme it interacts with is β-galactosidase . β-galactosidase rapidly hydrolyzes Fluorescein-digalactoside, first to fluorescein monogalactoside and then to highly fluorescent fluorescein . The fluorescence signal is proportional to the lacZ enzymatic activity .
Cellular Effects
Fluorescein-digalactoside enters viable cells and influences cell function by acting as a substrate for β-galactosidase . The hydrolysis of Fluorescein-digalactoside by β-galactosidase can be followed by the increase in either absorbance or fluorescence , indicating its impact on cellular metabolism.
Molecular Mechanism
The molecular mechanism of Fluorescein-digalactoside involves its sequential hydrolysis by β-galactosidase . This nonfluorescent compound is first hydrolyzed to fluorescein monogalactoside and then to highly fluorescent fluorescein . This process results in a fluorescence signal proportional to the enzymatic activity of lacZ .
Temporal Effects in Laboratory Settings
The effects of Fluorescein-digalactoside over time in laboratory settings are primarily related to its hydrolysis by β-galactosidase . The turnover rates of Fluorescein-digalactoside are slower than that of the common spectrophotometric galactosidase substrate . Fluorescence-based measurements can be several orders of magnitude more sensitive than absorption-based measurements .
Metabolic Pathways
Fluorescein-digalactoside is involved in the metabolic pathway of β-galactosidase . β-galactosidase rapidly hydrolyzes Fluorescein-digalactoside, leading to the production of fluorescein . This process can influence metabolic flux and metabolite levels.
Transport and Distribution
Fluorescein-digalactoside enters viable cells , indicating that it can be transported across cell membranes
准备方法
合成路线和反应条件
荧光素二-β-D-半乳糖苷可以通过荧光素与半乳糖衍生物的糖基化合成。 该反应通常涉及使用糖基供体,如半乳糖五乙酸酯,以及催化剂,如三氟化硼乙醚 。 该反应在无水溶剂(如二氯甲烷)中进行,在受控温度条件下进行,以确保高产率和纯度。
工业生产方法
荧光素二-β-D-半乳糖苷的工业生产遵循类似的合成路线,但在更大规模上进行。 该工艺涉及严格的纯化步骤,包括结晶和色谱法,以达到所需的产物质量。 然后将该化合物储存在特定条件下以保持其稳定性和活性 .
化学反应分析
反应类型
荧光素二-β-D-半乳糖苷主要经历由β-半乳糖苷酶催化的水解反应。 这种酶促反应导致半乳糖部分的顺序裂解,首先形成荧光素单半乳糖苷,然后形成荧光素 .
常用试剂和条件
水解反应需要存在β-半乳糖苷酶,并且通常在缓冲水溶液中在约 7.0 的最佳 pH 值下进行 。 通过测量荧光或吸光度的增加来监测反应。
主要产物
相似化合物的比较
类似化合物
荧光素二-β-D-葡萄糖苷: 结构类似,但含有葡萄糖而不是半乳糖.
邻硝基苯基-β-D-半乳糖苷: β-半乳糖苷酶的另一种底物,但产生比色产物而不是荧光产物.
氯丙嗪盐酸盐: 用于类似测定,但具有不同的化学性质和应用.
独特性
荧光素二-β-D-半乳糖苷因其对β-半乳糖苷酶的高度敏感性和特异性而独一无二,使其成为基于荧光的测定的理想底物。 它能够产生高度荧光的产物,从而可以灵敏地检测和定量酶活性,而比色底物无法做到这一点 .
生物活性
Fluorescein-digalactoside (FDG) is a fluorogenic substrate widely used in biological research, particularly for detecting β-galactosidase activity. This compound is crucial in various applications, including cell biology, microbiology, and cancer research. This article explores the biological activity of FDG, its mechanisms, applications, and relevant case studies.
Fluorescein-digalactoside is a nonfluorescent compound that becomes fluorescent upon hydrolysis by β-galactosidase. The hydrolysis process occurs in two steps:
- First Hydrolysis : FDG is hydrolyzed to fluorescein monogalactoside (FMG).
- Second Hydrolysis : FMG is further hydrolyzed to fluorescein, a highly fluorescent compound.
This reaction allows for the quantification of β-galactosidase activity through the measurement of fluorescence intensity, which correlates with enzymatic activity .
1. Detection of β-Galactosidase Activity
FDG is primarily used as a substrate for detecting β-galactosidase in various organisms, including bacteria and mammalian cells. The sensitivity of FDG makes it an ideal choice for flow cytometry and fluorescence microscopy. Studies have demonstrated its utility in:
- Identifying senescent cells : FDG can be used to detect senescence-associated β-galactosidase (SA-β-gal) activity, which is a marker for cellular aging .
- Analyzing recombinant proteins : Researchers utilize FDG to monitor the expression of β-galactosidase fusion proteins in genetically modified organisms .
2. Cellular Studies
FDG has been employed in various cellular studies to assess metabolic activity and cell viability. For instance:
- In cancer research , FDG has been utilized to study the metabolic differences between cancerous and normal cells by measuring β-galactosidase activity .
- In neurobiology , it has been used to investigate lysosomal storage diseases associated with deficient lysosomal β-galactosidase activity .
Case Study 1: Detection of Senescence in Human Cells
A study utilized FDG to quantify SA-β-gal activity in human foreskin fibroblast (Hs68) cells. The researchers developed a fluorimetric method that allowed for rapid quantification of senescence markers. The results indicated a significant increase in fluorescence intensity correlating with cellular senescence, demonstrating the effectiveness of FDG as a sensitive detection tool .
Case Study 2: Flow Cytometric Analysis
In another study, researchers employed FDG in flow cytometric analysis to sort viable cells expressing β-galactosidase. This method enabled the identification and isolation of specific cell populations based on their enzymatic activity, facilitating further studies on gene regulation at the single-cell level .
Comparative Analysis of Fluorogenic Substrates
The following table summarizes the characteristics of different fluorogenic substrates used for detecting β-galactosidase:
Substrate | Sensitivity | Application Area | Notes |
---|---|---|---|
Fluorescein-digalactoside | High | Cell biology, microbiology | Widely used; sensitive to enzymatic activity |
C12-FDG | Moderate | Animal cells | Requires cell permeabilization for yeast |
DDAO galactoside | High | Neurochemistry | Detects longer wavelengths; less autofluorescence interference |
属性
IUPAC Name |
3',6'-bis[[(2S,3R,4S,5R,6R)-3,4,5-trihydroxy-6-(hydroxymethyl)oxan-2-yl]oxy]spiro[2-benzofuran-3,9'-xanthene]-1-one | |
---|---|---|
Source | PubChem | |
URL | https://pubchem.ncbi.nlm.nih.gov | |
Description | Data deposited in or computed by PubChem | |
InChI |
InChI=1S/C32H32O15/c33-11-21-23(35)25(37)27(39)30(45-21)42-13-5-7-17-19(9-13)44-20-10-14(43-31-28(40)26(38)24(36)22(12-34)46-31)6-8-18(20)32(17)16-4-2-1-3-15(16)29(41)47-32/h1-10,21-28,30-31,33-40H,11-12H2/t21-,22-,23+,24+,25+,26+,27-,28-,30-,31-/m1/s1 | |
Source | PubChem | |
URL | https://pubchem.ncbi.nlm.nih.gov | |
Description | Data deposited in or computed by PubChem | |
InChI Key |
ZTOBILYWTYHOJB-WBCGDKOGSA-N | |
Source | PubChem | |
URL | https://pubchem.ncbi.nlm.nih.gov | |
Description | Data deposited in or computed by PubChem | |
Canonical SMILES |
C1=CC=C2C(=C1)C(=O)OC23C4=C(C=C(C=C4)OC5C(C(C(C(O5)CO)O)O)O)OC6=C3C=CC(=C6)OC7C(C(C(C(O7)CO)O)O)O | |
Source | PubChem | |
URL | https://pubchem.ncbi.nlm.nih.gov | |
Description | Data deposited in or computed by PubChem | |
Isomeric SMILES |
C1=CC=C2C(=C1)C(=O)OC23C4=C(C=C(C=C4)O[C@H]5[C@@H]([C@H]([C@H]([C@H](O5)CO)O)O)O)OC6=C3C=CC(=C6)O[C@H]7[C@@H]([C@H]([C@H]([C@H](O7)CO)O)O)O | |
Source | PubChem | |
URL | https://pubchem.ncbi.nlm.nih.gov | |
Description | Data deposited in or computed by PubChem | |
Molecular Formula |
C32H32O15 | |
Source | PubChem | |
URL | https://pubchem.ncbi.nlm.nih.gov | |
Description | Data deposited in or computed by PubChem | |
DSSTOX Substance ID |
DTXSID20170431 | |
Record name | Fluorescein-digalactoside | |
Source | EPA DSSTox | |
URL | https://comptox.epa.gov/dashboard/DTXSID20170431 | |
Description | DSSTox provides a high quality public chemistry resource for supporting improved predictive toxicology. | |
Molecular Weight |
656.6 g/mol | |
Source | PubChem | |
URL | https://pubchem.ncbi.nlm.nih.gov | |
Description | Data deposited in or computed by PubChem | |
CAS No. |
17817-20-8 | |
Record name | Fluorescein-digalactoside | |
Source | ChemIDplus | |
URL | https://pubchem.ncbi.nlm.nih.gov/substance/?source=chemidplus&sourceid=0017817208 | |
Description | ChemIDplus is a free, web search system that provides access to the structure and nomenclature authority files used for the identification of chemical substances cited in National Library of Medicine (NLM) databases, including the TOXNET system. | |
Record name | Fluorescein-digalactoside | |
Source | EPA DSSTox | |
URL | https://comptox.epa.gov/dashboard/DTXSID20170431 | |
Description | DSSTox provides a high quality public chemistry resource for supporting improved predictive toxicology. | |
Retrosynthesis Analysis
AI-Powered Synthesis Planning: Our tool employs the Template_relevance Pistachio, Template_relevance Bkms_metabolic, Template_relevance Pistachio_ringbreaker, Template_relevance Reaxys, Template_relevance Reaxys_biocatalysis model, leveraging a vast database of chemical reactions to predict feasible synthetic routes.
One-Step Synthesis Focus: Specifically designed for one-step synthesis, it provides concise and direct routes for your target compounds, streamlining the synthesis process.
Accurate Predictions: Utilizing the extensive PISTACHIO, BKMS_METABOLIC, PISTACHIO_RINGBREAKER, REAXYS, REAXYS_BIOCATALYSIS database, our tool offers high-accuracy predictions, reflecting the latest in chemical research and data.
Strategy Settings
Precursor scoring | Relevance Heuristic |
---|---|
Min. plausibility | 0.01 |
Model | Template_relevance |
Template Set | Pistachio/Bkms_metabolic/Pistachio_ringbreaker/Reaxys/Reaxys_biocatalysis |
Top-N result to add to graph | 6 |
Feasible Synthetic Routes
体外研究产品的免责声明和信息
请注意,BenchChem 上展示的所有文章和产品信息仅供信息参考。 BenchChem 上可购买的产品专为体外研究设计,这些研究在生物体外进行。体外研究,源自拉丁语 "in glass",涉及在受控实验室环境中使用细胞或组织进行的实验。重要的是要注意,这些产品没有被归类为药物或药品,他们没有得到 FDA 的批准,用于预防、治疗或治愈任何医疗状况、疾病或疾病。我们必须强调,将这些产品以任何形式引入人类或动物的身体都是法律严格禁止的。遵守这些指南对确保研究和实验的法律和道德标准的符合性至关重要。