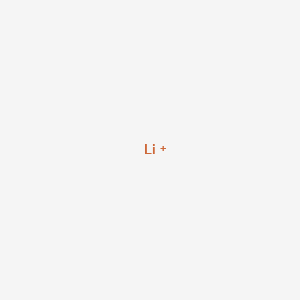
Lithium, ion (Li1+)
概述
描述
The lithium cation (Li⁺) is a positively charged ion of lithium, an alkali metal with the atomic number 3. Lithium is the lightest metal and the least dense solid element. It is highly reactive and flammable, and it is stored in mineral oil to prevent it from reacting with moisture and oxygen in the air. The lithium cation plays a crucial role in various applications, including medicine, chemistry, and industry .
准备方法
合成路线和反应条件
锂离子可以通过多种合成路线制备。一种常见的方法是使锂金属与水反应,生成氢氧化锂和氢气:
2Li+2H2O→2LiOH+H2
另一种方法是使碳酸锂与盐酸反应,生成氯化锂和二氧化碳:
Li2CO3+2HCl→2LiCl+CO2+H2O
工业生产方法
在工业上,锂是从锂辉石和锂云母等矿石中提取的。矿石首先被粉碎并加热以使其转化为更具反应性的形式。然后用硫酸处理以生产硫酸锂,然后进一步加工以获得碳酸锂。 然后将碳酸锂转化为氯化锂,氯化锂可以进行电解以生产锂金属和锂离子 .
化学反应分析
反应类型
锂离子经历各种类型的化学反应,包括:
氧化还原反应: 锂离子可以参与氧化还原反应,在其中它们被还原为锂金属。
取代反应: 锂离子由于其高反应性可以取代化合物中的其他阳离子。
络合反应: 锂离子可以与各种配体形成络合物,例如冠醚和穴醚。
常用试剂和条件
氧化还原: 锂离子可以使用强还原剂(如钠或钾)还原。
取代: 锂离子可以在适当条件下取代盐中的钠或钾阳离子。
络合: 锂离子在水或乙醇等极性溶剂中与配体形成稳定的络合物。
主要产物
氢氧化锂: 由锂离子与水反应生成。
碳酸锂: 由锂离子与碳酸根离子反应生成。
氯化锂: 由锂离子与氯离子反应生成.
科学研究应用
化学
在化学中,锂离子用于合成各种有机锂化合物,这些化合物是有机合成中重要的试剂。 它们也用于生产锂电池,锂电池广泛应用于便携式电子设备和电动汽车 .
生物学和医学
锂离子在医学上用作情绪稳定剂,用于治疗双相情感障碍。它们有助于调节情绪波动并降低双相情感障碍患者的自杀风险。 确切的作用机制尚不完全清楚,但据信涉及神经递质活性的调节和某些酶的抑制 .
工业
在工业中,锂离子用于生产高强度玻璃和陶瓷,以及润滑脂的生产。 它们也用于生产铝锂合金,这些合金由于其高强度重量比而用于航空航天工业 .
作用机制
锂离子在生物系统中的确切作用机制尚不完全清楚。 据信锂离子通过与神经元中一价或二价阳离子的转运相互作用来发挥作用。 它们还可以抑制糖原合酶激酶 3 和肌醇磷酸酶等酶,或调节谷氨酸受体 。这些相互作用有助于稳定情绪并减轻双相情感障碍的症状。
相似化合物的比较
类似化合物
- 钠离子 (Na⁺)
- 钾离子 (K⁺)
- 镁离子 (Mg²⁺)
比较
虽然锂离子与钠和钾等其他碱金属阳离子有一些相似之处,但它们也表现出独特的性质。例如,与钠和钾离子相比,锂离子具有更小的离子半径,这使得它们能够与某些配体和酶更强烈地相互作用。 此外,锂离子可以与不会轻易与钠或钾离子形成络合物的配体形成稳定的络合物 .
属性
IUPAC Name |
lithium(1+) | |
---|---|---|
Source | PubChem | |
URL | https://pubchem.ncbi.nlm.nih.gov | |
Description | Data deposited in or computed by PubChem | |
InChI |
InChI=1S/Li/q+1 | |
Source | PubChem | |
URL | https://pubchem.ncbi.nlm.nih.gov | |
Description | Data deposited in or computed by PubChem | |
InChI Key |
HBBGRARXTFLTSG-UHFFFAOYSA-N | |
Source | PubChem | |
URL | https://pubchem.ncbi.nlm.nih.gov | |
Description | Data deposited in or computed by PubChem | |
Canonical SMILES |
[Li+] | |
Source | PubChem | |
URL | https://pubchem.ncbi.nlm.nih.gov | |
Description | Data deposited in or computed by PubChem | |
Molecular Formula |
Li+ | |
Source | PubChem | |
URL | https://pubchem.ncbi.nlm.nih.gov | |
Description | Data deposited in or computed by PubChem | |
DSSTOX Substance ID |
DTXSID10169612 | |
Record name | Lithium cation | |
Source | EPA DSSTox | |
URL | https://comptox.epa.gov/dashboard/DTXSID10169612 | |
Description | DSSTox provides a high quality public chemistry resource for supporting improved predictive toxicology. | |
Molecular Weight |
7.0 g/mol | |
Source | PubChem | |
URL | https://pubchem.ncbi.nlm.nih.gov | |
Description | Data deposited in or computed by PubChem | |
Physical Description |
Solid | |
Record name | Lithium | |
Source | Human Metabolome Database (HMDB) | |
URL | http://www.hmdb.ca/metabolites/HMDB0005949 | |
Description | The Human Metabolome Database (HMDB) is a freely available electronic database containing detailed information about small molecule metabolites found in the human body. | |
Explanation | HMDB is offered to the public as a freely available resource. Use and re-distribution of the data, in whole or in part, for commercial purposes requires explicit permission of the authors and explicit acknowledgment of the source material (HMDB) and the original publication (see the HMDB citing page). We ask that users who download significant portions of the database cite the HMDB paper in any resulting publications. | |
Solubility |
Violent reaction | |
Record name | Lithium cation | |
Source | DrugBank | |
URL | https://www.drugbank.ca/drugs/DB01356 | |
Description | The DrugBank database is a unique bioinformatics and cheminformatics resource that combines detailed drug (i.e. chemical, pharmacological and pharmaceutical) data with comprehensive drug target (i.e. sequence, structure, and pathway) information. | |
Explanation | Creative Common's Attribution-NonCommercial 4.0 International License (http://creativecommons.org/licenses/by-nc/4.0/legalcode) | |
Mechanism of Action |
The precise mechanism of action of Li+ as a mood-stabilizing agent is currently unknown. It is possible that Li+ produces its effects by interacting with the transport of monovalent or divalent cations in neurons. An increasing number of scientists have come to the conclusion that the excitatory neurotransmitter glutamate is the key factor in understanding how lithium works. Lithium has been shown to change the inward and outward currents of glutamate receptors (especially GluR3), without a shift in reversal potential. Lithium has been found to exert a dual effect on glutamate receptors, acting to keep the amount of glutamate active between cells at a stable, healthy level, neither too much nor too little. It is postulated that too much glutamate in the space between neurons causes mania, and too little, depression. Another mechanism by which lithium might help to regulate mood include the non-competitive inhibition of an enzyme called inositol monophosphatase. Alternately lithium's action may be enhanced through the deactivation of the GSK-3B enzyme. The regulation of GSK-3B by lithium may affect the circadian clock. GSK-3 is known for phosphorylating and thus inactivating glycogen synthase. GSK-3B has also been implicated in the control of cellular response to damaged DNA. GSK-3 normally phosphorylates beta catenin, which leads to beta catenin degratation. When GSK-3 is inhibited, beta catenin increases and transgenic mice with overexpression of beta catenin express similar behaviour to mice treated with lithium. These results suggest that increase of beta catenin may be a possible pathway for the therapeutic action of lithium. | |
Record name | Lithium cation | |
Source | DrugBank | |
URL | https://www.drugbank.ca/drugs/DB01356 | |
Description | The DrugBank database is a unique bioinformatics and cheminformatics resource that combines detailed drug (i.e. chemical, pharmacological and pharmaceutical) data with comprehensive drug target (i.e. sequence, structure, and pathway) information. | |
Explanation | Creative Common's Attribution-NonCommercial 4.0 International License (http://creativecommons.org/licenses/by-nc/4.0/legalcode) | |
CAS No. |
17341-24-1, 7439-93-2 | |
Record name | Li1+ | |
Source | CAS Common Chemistry | |
URL | https://commonchemistry.cas.org/detail?cas_rn=17341-24-1 | |
Description | CAS Common Chemistry is an open community resource for accessing chemical information. Nearly 500,000 chemical substances from CAS REGISTRY cover areas of community interest, including common and frequently regulated chemicals, and those relevant to high school and undergraduate chemistry classes. This chemical information, curated by our expert scientists, is provided in alignment with our mission as a division of the American Chemical Society. | |
Explanation | The data from CAS Common Chemistry is provided under a CC-BY-NC 4.0 license, unless otherwise stated. | |
Record name | Lithium cation | |
Source | ChemIDplus | |
URL | https://pubchem.ncbi.nlm.nih.gov/substance/?source=chemidplus&sourceid=0017341241 | |
Description | ChemIDplus is a free, web search system that provides access to the structure and nomenclature authority files used for the identification of chemical substances cited in National Library of Medicine (NLM) databases, including the TOXNET system. | |
Record name | Lithium cation | |
Source | DrugBank | |
URL | https://www.drugbank.ca/drugs/DB01356 | |
Description | The DrugBank database is a unique bioinformatics and cheminformatics resource that combines detailed drug (i.e. chemical, pharmacological and pharmaceutical) data with comprehensive drug target (i.e. sequence, structure, and pathway) information. | |
Explanation | Creative Common's Attribution-NonCommercial 4.0 International License (http://creativecommons.org/licenses/by-nc/4.0/legalcode) | |
Record name | Lithium cation | |
Source | EPA DSSTox | |
URL | https://comptox.epa.gov/dashboard/DTXSID10169612 | |
Description | DSSTox provides a high quality public chemistry resource for supporting improved predictive toxicology. | |
Record name | LITHIUM CATION | |
Source | FDA Global Substance Registration System (GSRS) | |
URL | https://gsrs.ncats.nih.gov/ginas/app/beta/substances/8H8Z5UER66 | |
Description | The FDA Global Substance Registration System (GSRS) enables the efficient and accurate exchange of information on what substances are in regulated products. Instead of relying on names, which vary across regulatory domains, countries, and regions, the GSRS knowledge base makes it possible for substances to be defined by standardized, scientific descriptions. | |
Explanation | Unless otherwise noted, the contents of the FDA website (www.fda.gov), both text and graphics, are not copyrighted. They are in the public domain and may be republished, reprinted and otherwise used freely by anyone without the need to obtain permission from FDA. Credit to the U.S. Food and Drug Administration as the source is appreciated but not required. | |
Record name | Lithium | |
Source | Human Metabolome Database (HMDB) | |
URL | http://www.hmdb.ca/metabolites/HMDB0005949 | |
Description | The Human Metabolome Database (HMDB) is a freely available electronic database containing detailed information about small molecule metabolites found in the human body. | |
Explanation | HMDB is offered to the public as a freely available resource. Use and re-distribution of the data, in whole or in part, for commercial purposes requires explicit permission of the authors and explicit acknowledgment of the source material (HMDB) and the original publication (see the HMDB citing page). We ask that users who download significant portions of the database cite the HMDB paper in any resulting publications. | |
Melting Point |
190 °C | |
Record name | Lithium cation | |
Source | DrugBank | |
URL | https://www.drugbank.ca/drugs/DB01356 | |
Description | The DrugBank database is a unique bioinformatics and cheminformatics resource that combines detailed drug (i.e. chemical, pharmacological and pharmaceutical) data with comprehensive drug target (i.e. sequence, structure, and pathway) information. | |
Explanation | Creative Common's Attribution-NonCommercial 4.0 International License (http://creativecommons.org/licenses/by-nc/4.0/legalcode) | |
Record name | Lithium | |
Source | Human Metabolome Database (HMDB) | |
URL | http://www.hmdb.ca/metabolites/HMDB0005949 | |
Description | The Human Metabolome Database (HMDB) is a freely available electronic database containing detailed information about small molecule metabolites found in the human body. | |
Explanation | HMDB is offered to the public as a freely available resource. Use and re-distribution of the data, in whole or in part, for commercial purposes requires explicit permission of the authors and explicit acknowledgment of the source material (HMDB) and the original publication (see the HMDB citing page). We ask that users who download significant portions of the database cite the HMDB paper in any resulting publications. | |
Synthesis routes and methods I
Procedure details
Synthesis routes and methods II
Procedure details
Synthesis routes and methods III
Procedure details
Synthesis routes and methods IV
Procedure details
Synthesis routes and methods V
Procedure details
体外研究产品的免责声明和信息
请注意,BenchChem 上展示的所有文章和产品信息仅供信息参考。 BenchChem 上可购买的产品专为体外研究设计,这些研究在生物体外进行。体外研究,源自拉丁语 "in glass",涉及在受控实验室环境中使用细胞或组织进行的实验。重要的是要注意,这些产品没有被归类为药物或药品,他们没有得到 FDA 的批准,用于预防、治疗或治愈任何医疗状况、疾病或疾病。我们必须强调,将这些产品以任何形式引入人类或动物的身体都是法律严格禁止的。遵守这些指南对确保研究和实验的法律和道德标准的符合性至关重要。