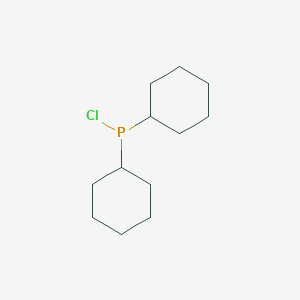
二氯环己基膦
描述
Chlorodicyclohexylphosphine, also known as Dicyclohexylchlorophosphine, Dicyclohexylphosphine chloride, or Dicyclohexylphosphinous chloride, is a chemical compound with the linear formula (C6H11)2PCl . It has a molecular weight of 232.73 .
Synthesis Analysis
Chlorodicyclohexylphosphine is used as a pharmaceutical intermediate . It is also used in the synthesis of various phosphines . For instance, it can be used as a reactant in the synthesis of 1,2-Bis(dicyclohexylphosphinoxy)ethane ligand by reacting with ethylene glycol in the presence of triethylamine via Michaelis−Arbuzov type rearrangements .
Molecular Structure Analysis
The molecular structure of Chlorodicyclohexylphosphine is represented by the linear formula (C6H11)2PCl . It has a molecular weight of 232.73 .
Chemical Reactions Analysis
Chlorodicyclohexylphosphine is used in various chemical reactions. It is particularly useful in the synthesis of various phosphines . It can be used as a reactant in the synthesis of 1,2-Bis(dicyclohexylphosphinoxy)ethane ligand by reacting with ethylene glycol in the presence of triethylamine via Michaelis−Arbuzov type rearrangements .
Physical And Chemical Properties Analysis
Chlorodicyclohexylphosphine has a boiling point of 297.5±7.0 °C at 760 mmHg . Its vapour pressure is 0.0±0.6 mmHg at 25°C . The enthalpy of vaporization is 51.6±3.0 kJ/mol . The flash point is 133.7±18.2 °C .
科学研究应用
Synthesis of Ligands
Chlorodicyclohexylphosphine can be used as a reactant in the synthesis of various ligands. For instance, it can be used to synthesize the 1,2-Bis (dicyclohexylphosphinoxy)ethane ligand by reacting with ethylene glycol in the presence of triethylamine via Michaelis−Arbuzov type rearrangements .
Preparation of Monosulfide Ligands
Another application of Chlorodicyclohexylphosphine is in the preparation of 1,1,2,2-tetracyclohexyldiphosphine monosulfide ligand . This is achieved by treating Chlorodicyclohexylphosphine with LiS .
Production of Phosphine Oxide
Chlorodicyclohexylphosphine can also be used as a starting material for the preparation of dicyclohexylphosphine oxide .
Synthesis of Phosphino Substituted N-Aryl Pyrroles
Chlorodicyclohexylphosphine can be used in the synthesis of phosphino substituted N-aryl pyrroles .
Production of Di-tert-butyl ((dicyclohexylphosphino)methyl)phosphine
Another application of Chlorodicyclohexylphosphine is in the production of di-tert-butyl ((dicyclohexylphosphino)methyl)phosphine .
Synthesis of Dicyclohexylcyclopentylphosphine
Chlorodicyclohexylphosphine can also be used in the synthesis of dicyclohexylcyclopentylphosphine .
安全和危害
作用机制
Target of Action
Chlorodicyclohexylphosphine is primarily used as a reactant in the synthesis of various organic phosphine ligands . These ligands can then interact with a variety of targets, depending on their specific structure and properties.
Mode of Action
The compound interacts with its targets through a variety of chemical reactions. For instance, it can be used in the synthesis of 1,2-Bis(dicyclohexylphosphinoxy)ethane ligand by reacting with ethylene glycol in the presence of triethylamine via Michaelis−Arbuzov type rearrangements .
Biochemical Pathways
The exact biochemical pathways affected by Chlorodicyclohexylphosphine depend on the specific ligands that are synthesized using it. It’s worth noting that the compound is often used in reactions such as buchwald-hartwig cross coupling, heck reaction, hiyama coupling, negishi coupling, sonogashira coupling, stille coupling, and suzuki-miyaura coupling .
Result of Action
The molecular and cellular effects of Chlorodicyclohexylphosphine’s action would depend on the specific ligands synthesized using it and their subsequent interactions with their targets. For example, it can be used to synthesize 1,1,2,2-tetracyclohexyldiphosphine monosulfide ligand by treating with LiS .
Action Environment
The action, efficacy, and stability of Chlorodicyclohexylphosphine can be influenced by various environmental factors. For instance, its reactions should be carried out under inert gas (nitrogen or Argon) at 2-8°C . Additionally, it’s sensitive to air and moisture .
属性
IUPAC Name |
chloro(dicyclohexyl)phosphane | |
---|---|---|
Source | PubChem | |
URL | https://pubchem.ncbi.nlm.nih.gov | |
Description | Data deposited in or computed by PubChem | |
InChI |
InChI=1S/C12H22ClP/c13-14(11-7-3-1-4-8-11)12-9-5-2-6-10-12/h11-12H,1-10H2 | |
Source | PubChem | |
URL | https://pubchem.ncbi.nlm.nih.gov | |
Description | Data deposited in or computed by PubChem | |
InChI Key |
AKJFBIZAEPTXIL-UHFFFAOYSA-N | |
Source | PubChem | |
URL | https://pubchem.ncbi.nlm.nih.gov | |
Description | Data deposited in or computed by PubChem | |
Canonical SMILES |
C1CCC(CC1)P(C2CCCCC2)Cl | |
Source | PubChem | |
URL | https://pubchem.ncbi.nlm.nih.gov | |
Description | Data deposited in or computed by PubChem | |
Molecular Formula |
C12H22ClP | |
Source | PubChem | |
URL | https://pubchem.ncbi.nlm.nih.gov | |
Description | Data deposited in or computed by PubChem | |
DSSTOX Substance ID |
DTXSID10370140 | |
Record name | Chlorodicyclohexylphosphine | |
Source | EPA DSSTox | |
URL | https://comptox.epa.gov/dashboard/DTXSID10370140 | |
Description | DSSTox provides a high quality public chemistry resource for supporting improved predictive toxicology. | |
Molecular Weight |
232.73 g/mol | |
Source | PubChem | |
URL | https://pubchem.ncbi.nlm.nih.gov | |
Description | Data deposited in or computed by PubChem | |
Product Name |
Chlorodicyclohexylphosphine | |
CAS RN |
16523-54-9 | |
Record name | Chlorodicyclohexylphosphine | |
Source | CAS Common Chemistry | |
URL | https://commonchemistry.cas.org/detail?cas_rn=16523-54-9 | |
Description | CAS Common Chemistry is an open community resource for accessing chemical information. Nearly 500,000 chemical substances from CAS REGISTRY cover areas of community interest, including common and frequently regulated chemicals, and those relevant to high school and undergraduate chemistry classes. This chemical information, curated by our expert scientists, is provided in alignment with our mission as a division of the American Chemical Society. | |
Explanation | The data from CAS Common Chemistry is provided under a CC-BY-NC 4.0 license, unless otherwise stated. | |
Record name | Chlorodicyclohexylphosphine | |
Source | EPA DSSTox | |
URL | https://comptox.epa.gov/dashboard/DTXSID10370140 | |
Description | DSSTox provides a high quality public chemistry resource for supporting improved predictive toxicology. | |
Synthesis routes and methods I
Procedure details
Synthesis routes and methods II
Procedure details
Retrosynthesis Analysis
AI-Powered Synthesis Planning: Our tool employs the Template_relevance Pistachio, Template_relevance Bkms_metabolic, Template_relevance Pistachio_ringbreaker, Template_relevance Reaxys, Template_relevance Reaxys_biocatalysis model, leveraging a vast database of chemical reactions to predict feasible synthetic routes.
One-Step Synthesis Focus: Specifically designed for one-step synthesis, it provides concise and direct routes for your target compounds, streamlining the synthesis process.
Accurate Predictions: Utilizing the extensive PISTACHIO, BKMS_METABOLIC, PISTACHIO_RINGBREAKER, REAXYS, REAXYS_BIOCATALYSIS database, our tool offers high-accuracy predictions, reflecting the latest in chemical research and data.
Strategy Settings
Precursor scoring | Relevance Heuristic |
---|---|
Min. plausibility | 0.01 |
Model | Template_relevance |
Template Set | Pistachio/Bkms_metabolic/Pistachio_ringbreaker/Reaxys/Reaxys_biocatalysis |
Top-N result to add to graph | 6 |
Feasible Synthetic Routes
Q & A
Q1: What are the main applications of Chlorodicyclohexylphosphine in chemical synthesis?
A1: Chlorodicyclohexylphosphine (ClP(C6H11)2) serves as a versatile reagent for synthesizing various organophosphorus compounds, particularly phosphine ligands. These ligands play a crucial role in transition metal catalysis, enabling numerous C-C and C-N bond-forming reactions. [, , , , ]
Q2: How does the structure of Chlorodicyclohexylphosphine influence its reactivity?
A2: The presence of two bulky cyclohexyl groups on the phosphorus atom imparts steric hindrance, influencing the reactivity and selectivity of Chlorodicyclohexylphosphine. This steric bulk can enhance the activity and stability of metal complexes formed with this ligand. For instance, in palladium-catalyzed Suzuki cross-coupling reactions, complexes containing bulky phosphine ligands, like those derived from Chlorodicyclohexylphosphine, exhibited remarkable catalytic activity, achieving high turnover frequencies. [, ]
Q3: Are there any alternative synthetic routes for Chlorodicyclohexylphosphine that offer advantages over conventional methods?
A3: Yes, an improved synthesis of functionalized biphenyl-based phosphine ligands, often derived from Chlorodicyclohexylphosphine, involves reacting arylmagnesium halides with benzyne followed by the addition of Chlorodicyclohexylphosphine. This one-pot procedure offers significant cost and time advantages compared to traditional multi-step syntheses. Additionally, directly using Chlorodicyclohexylphosphine prepared from phosphorus trichloride (PCl3) and cyclohexylmagnesium chloride without further purification can further reduce costs. []
Q4: Can Chlorodicyclohexylphosphine be incorporated into chiral ligands?
A4: Yes, Chlorodicyclohexylphosphine can be incorporated into chiral ferrocenylaminophosphine ligands. These ligands are synthesized by reacting a chiral ferrocene template with Chlorodicyclohexylphosphine, creating a chiral center at the phosphorus atom. These chiral ligands show promise in asymmetric catalysis, offering enantioselective control in reactions like the hydrogenation of prochiral substrates. []
Q5: What safety precautions should be taken when handling Chlorodicyclohexylphosphine?
A5: Chlorodicyclohexylphosphine should be handled with caution as it can be irritating to the respiratory tract, skin, and eyes. It may also be harmful if swallowed. While it exhibits low reactivity towards air oxidation, storage under nitrogen in a desiccator is recommended. It's essential to avoid contact with strong oxidizing agents. Long-term effects of exposure are currently unknown. []
Q6: Are there any specific analytical techniques used to characterize Chlorodicyclohexylphosphine and its derivatives?
A6: Nuclear Magnetic Resonance (NMR) spectroscopy, particularly 1H and 31P NMR, serves as a primary tool for analyzing the purity and structure of Chlorodicyclohexylphosphine and its derivatives. [, ] Additionally, infrared (IR) spectroscopy can provide valuable information about the functional groups present in these compounds. []
体外研究产品的免责声明和信息
请注意,BenchChem 上展示的所有文章和产品信息仅供信息参考。 BenchChem 上可购买的产品专为体外研究设计,这些研究在生物体外进行。体外研究,源自拉丁语 "in glass",涉及在受控实验室环境中使用细胞或组织进行的实验。重要的是要注意,这些产品没有被归类为药物或药品,他们没有得到 FDA 的批准,用于预防、治疗或治愈任何医疗状况、疾病或疾病。我们必须强调,将这些产品以任何形式引入人类或动物的身体都是法律严格禁止的。遵守这些指南对确保研究和实验的法律和道德标准的符合性至关重要。