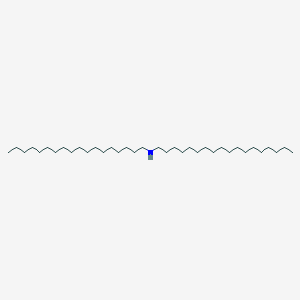
Dioctadecylamine
描述
C₃₆H₇₅N . It is a fatty amine derivative, characterized by its long hydrocarbon chains, which contribute to its hydrophobic properties. This compound is known for its ability to self-organize into plate-like structures in aqueous solutions .
准备方法
Synthetic Routes and Reaction Conditions: Dioctadecylamine can be synthesized through the reaction of octadecylamine with octadecyl chloride in the presence of a base such as sodium hydroxide. The reaction typically occurs under reflux conditions, and the product is purified through recrystallization .
Industrial Production Methods: In industrial settings, this compound is produced by the hydrogenation of octadecyl nitrile. This process involves the reduction of the nitrile group to an amine group using hydrogen gas in the presence of a catalyst such as nickel or palladium. The reaction is carried out at elevated temperatures and pressures to achieve high yields .
Types of Reactions:
Oxidation: this compound can undergo oxidation reactions to form corresponding amides or nitriles.
Reduction: It can be reduced to form primary amines.
Substitution: this compound can participate in nucleophilic substitution reactions, where the amine group is replaced by other functional groups.
Common Reagents and Conditions:
Oxidation: Common oxidizing agents include potassium permanganate and hydrogen peroxide.
Reduction: Reducing agents such as lithium aluminum hydride or hydrogen gas in the presence of a catalyst are used.
Substitution: Reagents like alkyl halides or acyl chlorides are used in substitution reactions.
Major Products:
Oxidation: Amides or nitriles.
Reduction: Primary amines.
Substitution: Various substituted amines depending on the reagents used.
科学研究应用
Dioctadecylamine has a wide range of applications in scientific research:
Chemistry: It is used as a reagent in the synthesis of various organic compounds, including lipids and polymers.
Biology: this compound is utilized in the preparation of liposomes and cationic lipids, which are essential for drug delivery systems.
Medicine: It is used in the formulation of lipid-based drug delivery systems, enhancing the bioavailability of hydrophobic drugs.
Industry: this compound is employed in the production of surfactants, lubricants, and corrosion inhibitors.
作用机制
Dioctadecylamine exerts its effects primarily through its hydrophobic interactions. The long hydrocarbon chains allow it to interact with lipid bilayers, making it an effective component in liposome formation. It can also form complexes with metal ions, acting as a chelating agent. The molecular targets and pathways involved include lipid membranes and metal ions .
相似化合物的比较
Octadecylamine: A primary amine with a single octadecyl group.
Didodecylamine: A secondary amine with two dodecyl groups.
Hexadecylamine: A primary amine with a single hexadecyl group.
Comparison: Dioctadecylamine is unique due to its two long octadecyl chains, which provide greater hydrophobicity and the ability to form more stable structures in aqueous solutions compared to its counterparts. This makes it particularly useful in applications requiring strong hydrophobic interactions, such as in the formation of liposomes and surfactants .
属性
IUPAC Name |
N-octadecyloctadecan-1-amine | |
---|---|---|
Source | PubChem | |
URL | https://pubchem.ncbi.nlm.nih.gov | |
Description | Data deposited in or computed by PubChem | |
InChI |
InChI=1S/C36H75N/c1-3-5-7-9-11-13-15-17-19-21-23-25-27-29-31-33-35-37-36-34-32-30-28-26-24-22-20-18-16-14-12-10-8-6-4-2/h37H,3-36H2,1-2H3 | |
Source | PubChem | |
URL | https://pubchem.ncbi.nlm.nih.gov | |
Description | Data deposited in or computed by PubChem | |
InChI Key |
HKUFIYBZNQSHQS-UHFFFAOYSA-N | |
Source | PubChem | |
URL | https://pubchem.ncbi.nlm.nih.gov | |
Description | Data deposited in or computed by PubChem | |
Canonical SMILES |
CCCCCCCCCCCCCCCCCCNCCCCCCCCCCCCCCCCCC | |
Source | PubChem | |
URL | https://pubchem.ncbi.nlm.nih.gov | |
Description | Data deposited in or computed by PubChem | |
Molecular Formula |
C36H75N | |
Source | PubChem | |
URL | https://pubchem.ncbi.nlm.nih.gov | |
Description | Data deposited in or computed by PubChem | |
DSSTOX Substance ID |
DTXSID8059425 | |
Record name | 1-Octadecanamine, N-octadecyl- | |
Source | EPA DSSTox | |
URL | https://comptox.epa.gov/dashboard/DTXSID8059425 | |
Description | DSSTox provides a high quality public chemistry resource for supporting improved predictive toxicology. | |
Molecular Weight |
522.0 g/mol | |
Source | PubChem | |
URL | https://pubchem.ncbi.nlm.nih.gov | |
Description | Data deposited in or computed by PubChem | |
CAS No. |
112-99-2 | |
Record name | Dioctadecylamine | |
Source | CAS Common Chemistry | |
URL | https://commonchemistry.cas.org/detail?cas_rn=112-99-2 | |
Description | CAS Common Chemistry is an open community resource for accessing chemical information. Nearly 500,000 chemical substances from CAS REGISTRY cover areas of community interest, including common and frequently regulated chemicals, and those relevant to high school and undergraduate chemistry classes. This chemical information, curated by our expert scientists, is provided in alignment with our mission as a division of the American Chemical Society. | |
Explanation | The data from CAS Common Chemistry is provided under a CC-BY-NC 4.0 license, unless otherwise stated. | |
Record name | Dioctadecylamine | |
Source | ChemIDplus | |
URL | https://pubchem.ncbi.nlm.nih.gov/substance/?source=chemidplus&sourceid=0000112992 | |
Description | ChemIDplus is a free, web search system that provides access to the structure and nomenclature authority files used for the identification of chemical substances cited in National Library of Medicine (NLM) databases, including the TOXNET system. | |
Record name | 1-Octadecanamine, N-octadecyl- | |
Source | EPA Chemicals under the TSCA | |
URL | https://www.epa.gov/chemicals-under-tsca | |
Description | EPA Chemicals under the Toxic Substances Control Act (TSCA) collection contains information on chemicals and their regulations under TSCA, including non-confidential content from the TSCA Chemical Substance Inventory and Chemical Data Reporting. | |
Record name | 1-Octadecanamine, N-octadecyl- | |
Source | EPA DSSTox | |
URL | https://comptox.epa.gov/dashboard/DTXSID8059425 | |
Description | DSSTox provides a high quality public chemistry resource for supporting improved predictive toxicology. | |
Record name | Dioctadecylamine | |
Source | European Chemicals Agency (ECHA) | |
URL | https://echa.europa.eu/substance-information/-/substanceinfo/100.003.655 | |
Description | The European Chemicals Agency (ECHA) is an agency of the European Union which is the driving force among regulatory authorities in implementing the EU's groundbreaking chemicals legislation for the benefit of human health and the environment as well as for innovation and competitiveness. | |
Explanation | Use of the information, documents and data from the ECHA website is subject to the terms and conditions of this Legal Notice, and subject to other binding limitations provided for under applicable law, the information, documents and data made available on the ECHA website may be reproduced, distributed and/or used, totally or in part, for non-commercial purposes provided that ECHA is acknowledged as the source: "Source: European Chemicals Agency, http://echa.europa.eu/". Such acknowledgement must be included in each copy of the material. ECHA permits and encourages organisations and individuals to create links to the ECHA website under the following cumulative conditions: Links can only be made to webpages that provide a link to the Legal Notice page. | |
Record name | DISTEARYLAMINE | |
Source | FDA Global Substance Registration System (GSRS) | |
URL | https://gsrs.ncats.nih.gov/ginas/app/beta/substances/569INV7SP8 | |
Description | The FDA Global Substance Registration System (GSRS) enables the efficient and accurate exchange of information on what substances are in regulated products. Instead of relying on names, which vary across regulatory domains, countries, and regions, the GSRS knowledge base makes it possible for substances to be defined by standardized, scientific descriptions. | |
Explanation | Unless otherwise noted, the contents of the FDA website (www.fda.gov), both text and graphics, are not copyrighted. They are in the public domain and may be republished, reprinted and otherwise used freely by anyone without the need to obtain permission from FDA. Credit to the U.S. Food and Drug Administration as the source is appreciated but not required. | |
Synthesis routes and methods
Procedure details
Retrosynthesis Analysis
AI-Powered Synthesis Planning: Our tool employs the Template_relevance Pistachio, Template_relevance Bkms_metabolic, Template_relevance Pistachio_ringbreaker, Template_relevance Reaxys, Template_relevance Reaxys_biocatalysis model, leveraging a vast database of chemical reactions to predict feasible synthetic routes.
One-Step Synthesis Focus: Specifically designed for one-step synthesis, it provides concise and direct routes for your target compounds, streamlining the synthesis process.
Accurate Predictions: Utilizing the extensive PISTACHIO, BKMS_METABOLIC, PISTACHIO_RINGBREAKER, REAXYS, REAXYS_BIOCATALYSIS database, our tool offers high-accuracy predictions, reflecting the latest in chemical research and data.
Strategy Settings
Precursor scoring | Relevance Heuristic |
---|---|
Min. plausibility | 0.01 |
Model | Template_relevance |
Template Set | Pistachio/Bkms_metabolic/Pistachio_ringbreaker/Reaxys/Reaxys_biocatalysis |
Top-N result to add to graph | 6 |
Feasible Synthetic Routes
体外研究产品的免责声明和信息
请注意,BenchChem 上展示的所有文章和产品信息仅供信息参考。 BenchChem 上可购买的产品专为体外研究设计,这些研究在生物体外进行。体外研究,源自拉丁语 "in glass",涉及在受控实验室环境中使用细胞或组织进行的实验。重要的是要注意,这些产品没有被归类为药物或药品,他们没有得到 FDA 的批准,用于预防、治疗或治愈任何医疗状况、疾病或疾病。我们必须强调,将这些产品以任何形式引入人类或动物的身体都是法律严格禁止的。遵守这些指南对确保研究和实验的法律和道德标准的符合性至关重要。