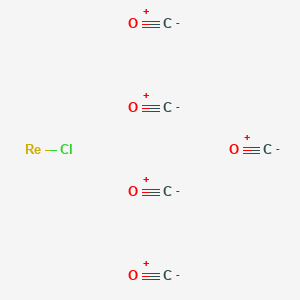
五羰基氯代铼(I)
描述
Pentacarbonylchlororhenium(I) is an organometallic compound with the molecular formula Re(CO)5Cl . It is used in the study of charge-transfer processes . It appears as a white to off-white powder .
Molecular Structure Analysis
The molecular structure of Pentacarbonylchlororhenium(I) is represented by the formula Re(CO)5Cl . This indicates that the molecule consists of one rhenium atom, five carbonyl groups, and one chloride atom.Physical And Chemical Properties Analysis
Pentacarbonylchlororhenium(I) is a white to off-white powder . Its molecular weight is 361.71 .科学研究应用
发光性质和X射线晶体结构:
- 五羰基氯代铼与2,7-二甲基-1,8-萘啶反应形成发光配合物,在溶液中表现出配体中心发射,在固态中表现出3MLCT发射。这种配合物在空气中稳定,但在某些条件下显示出配体的不稳定性 (Andrews, Laye, & Pope, 2009)。
三羰基配合物的形成:
- 五羰基卤代铼(I)配合物与2,2′:6′,2″-三吡啶形成稳定的八面体三羰基配合物。这些配合物在溶液中表现出流动性行为,其旋转的能垒由Abel等人(1993年)估计。
有机发光二极管(OLEDs):
- 中性铼(I)三羰基配合物,包括从五羰基氯代铼(I)衍生的配合物,由于其磷光发射、优异的热稳定性和光化学稳定性,具有在OLEDs中的潜力 (Zhao et al., 2016)。
生物应用:
- 可从五羰基氯代铼(I)衍生的发光铼(I)三羰基多吡啶配合物已被开发用于作为细胞成像试剂、抗癌药物和抗菌剂。这些配合物具有多样的光物理性质,并具有在光动力疗法中使用的潜力 (Lee, Leung, & Lo, 2017)。
配位化学中的光物理性质:
- 五羰基氯代铼(I)在形成铼(I)三羰基基团中起关键作用,这对于电子转移、能量转移和光解离过程至关重要。这些配合物在CO2还原、DNA裂解以及作为生物标记物中有应用 (Coleman, Brennan, Vos, & Pryce, 2008)。
表面位点探测:
- 可从五羰基氯代铼(I)衍生的铼羰基配合物已被用于探测TiO2表面位点。这些研究为我们提供了关于表面反应和表面铼三羰基和四羰基的形成的见解 (Lobo-Lapidus & Gates, 2010)。
安全和危害
作用机制
Target of Action
Pentacarbonylchlororhenium, also known as Rhenium pentacarbonyl chloride or Pentacarbonylchlororhenium(I), is primarily used to study π-electron deficient complexes between tricarbonylhalorhenium and diimine ligands . It is also used in the preparation of a series of mixed-valence compounds of rhenium and iron . These compounds find application in the study of charge-transfer processes .
Mode of Action
It is known that it is used to study π-electron deficient complexes between tricarbonylhalorhenium and diimine ligands . This suggests that it may interact with these targets to facilitate the study of charge-transfer processes .
Biochemical Pathways
Given its use in the study of charge-transfer processes , it can be inferred that it may influence pathways related to electron transfer.
Result of Action
Its use in the study of charge-transfer processes suggests that it may influence electron transfer within cells, potentially affecting cellular energy production and other processes.
Action Environment
It is classified as a dangerous good for transport , indicating that certain precautions must be taken when handling and storing this compound to ensure its stability and safety.
属性
IUPAC Name |
carbon monoxide;chlororhenium | |
---|---|---|
Source | PubChem | |
URL | https://pubchem.ncbi.nlm.nih.gov | |
Description | Data deposited in or computed by PubChem | |
InChI |
InChI=1S/5CO.ClH.Re/c5*1-2;;/h;;;;;1H;/q;;;;;;+1/p-1 | |
Source | PubChem | |
URL | https://pubchem.ncbi.nlm.nih.gov | |
Description | Data deposited in or computed by PubChem | |
InChI Key |
JQUUAHKBIXPQAP-UHFFFAOYSA-M | |
Source | PubChem | |
URL | https://pubchem.ncbi.nlm.nih.gov | |
Description | Data deposited in or computed by PubChem | |
Canonical SMILES |
[C-]#[O+].[C-]#[O+].[C-]#[O+].[C-]#[O+].[C-]#[O+].Cl[Re] | |
Source | PubChem | |
URL | https://pubchem.ncbi.nlm.nih.gov | |
Description | Data deposited in or computed by PubChem | |
Molecular Formula |
C5ClO5Re | |
Source | PubChem | |
URL | https://pubchem.ncbi.nlm.nih.gov | |
Description | Data deposited in or computed by PubChem | |
DSSTOX Substance ID |
DTXSID30161512 | |
Record name | Pentacarbonylchlororhenium | |
Source | EPA DSSTox | |
URL | https://comptox.epa.gov/dashboard/DTXSID30161512 | |
Description | DSSTox provides a high quality public chemistry resource for supporting improved predictive toxicology. | |
Molecular Weight |
361.71 g/mol | |
Source | PubChem | |
URL | https://pubchem.ncbi.nlm.nih.gov | |
Description | Data deposited in or computed by PubChem | |
Product Name |
Pentacarbonylchlororhenium | |
CAS RN |
14099-01-5 | |
Record name | Pentacarbonylchlororhenium | |
Source | CAS Common Chemistry | |
URL | https://commonchemistry.cas.org/detail?cas_rn=14099-01-5 | |
Description | CAS Common Chemistry is an open community resource for accessing chemical information. Nearly 500,000 chemical substances from CAS REGISTRY cover areas of community interest, including common and frequently regulated chemicals, and those relevant to high school and undergraduate chemistry classes. This chemical information, curated by our expert scientists, is provided in alignment with our mission as a division of the American Chemical Society. | |
Explanation | The data from CAS Common Chemistry is provided under a CC-BY-NC 4.0 license, unless otherwise stated. | |
Record name | Pentacarbonylchlororhenium | |
Source | EPA DSSTox | |
URL | https://comptox.epa.gov/dashboard/DTXSID30161512 | |
Description | DSSTox provides a high quality public chemistry resource for supporting improved predictive toxicology. | |
Record name | Pentacarbonylchlororhenium | |
Source | European Chemicals Agency (ECHA) | |
URL | https://echa.europa.eu/substance-information/-/substanceinfo/100.034.484 | |
Description | The European Chemicals Agency (ECHA) is an agency of the European Union which is the driving force among regulatory authorities in implementing the EU's groundbreaking chemicals legislation for the benefit of human health and the environment as well as for innovation and competitiveness. | |
Explanation | Use of the information, documents and data from the ECHA website is subject to the terms and conditions of this Legal Notice, and subject to other binding limitations provided for under applicable law, the information, documents and data made available on the ECHA website may be reproduced, distributed and/or used, totally or in part, for non-commercial purposes provided that ECHA is acknowledged as the source: "Source: European Chemicals Agency, http://echa.europa.eu/". Such acknowledgement must be included in each copy of the material. ECHA permits and encourages organisations and individuals to create links to the ECHA website under the following cumulative conditions: Links can only be made to webpages that provide a link to the Legal Notice page. | |
Q & A
Q1: What is the structure of Rhenium pentacarbonyl chloride and are there any notable structural features?
A1: Rhenium pentacarbonyl chloride (Re(CO)5Cl) is a discrete monomeric unit with a central Rhenium atom bonded to five carbonyl ligands and one chlorine atom. While the molecule possesses required mirror symmetry, it exhibits non-crystallographic 4mm symmetry. [] The crystal structure itself belongs to the orthorhombic Pnma space group. []
Q2: How does the structure of Rhenium pentacarbonyl chloride relate to its vibrational spectra?
A2: The vibrational spectra of Rhenium pentacarbonyl chloride, particularly in the far-IR and Raman regions, can be assigned by comparing it to the established assignments of the analogous bromide compound. [] Notably, the pressure-induced changes in the vibrational modes of Rhenium pentacarbonyl chloride, specifically the crossover between A1, ν6, ν(Re—I) and E, ν20, δ(CReC) modes, help confirm aspects of these spectral assignments. []
Q3: What are the catalytic applications of Rhenium pentacarbonyl chloride?
A3: Rhenium pentacarbonyl chloride, when combined with ethylaluminium dichloride, forms a highly active and long-lived homogeneous catalyst for olefin metathesis reactions. [] This catalyst effectively facilitates the metathesis of both terminal and internal olefins. [] Evidence suggests that the reaction mechanism involves a propyl carbene initiation step. []
Q4: Can you elaborate on the interaction of Rhenium pentacarbonyl chloride with porphyrins?
A4: When reacted with octaethylporphine (OEP) in a 2:1 molar ratio under specific conditions, Rhenium pentacarbonyl chloride can form two distinct products: dirhenium octaethylporphine (OEP(Re(CO)3)2) and a salt-type complex. [] This salt-type complex consists of an octaethylporphinium monocation [(H3OEP)+] and a novel anionic halogenocarbonyl rhenium(I) species [(Re2(CO)6Cl3)-]. [] The anionic species in this complex exhibits an unusual structure. []
Q5: Are there any known alternative compounds to Rhenium pentacarbonyl chloride in its catalytic applications?
A5: While the provided research papers do not directly compare Rhenium pentacarbonyl chloride to alternative catalysts for olefin metathesis, the field of olefin metathesis employs a variety of catalytic systems. Further research is needed to compare the performance, cost, and impact of these alternatives to Rhenium pentacarbonyl chloride. []
Q6: What are the future directions for research on Rhenium pentacarbonyl chloride?
A6: Further research on Rhenium pentacarbonyl chloride could explore its potential in various catalytic reactions beyond olefin metathesis. Investigating its coordination chemistry with a wider range of ligands, as exemplified by its interaction with H-spirophosphorane ligands [], could uncover new applications. Additionally, exploring its reactivity under different conditions and studying its detailed mechanistic pathways in various reactions will enhance our understanding and potential uses of this compound.
体外研究产品的免责声明和信息
请注意,BenchChem 上展示的所有文章和产品信息仅供信息参考。 BenchChem 上可购买的产品专为体外研究设计,这些研究在生物体外进行。体外研究,源自拉丁语 "in glass",涉及在受控实验室环境中使用细胞或组织进行的实验。重要的是要注意,这些产品没有被归类为药物或药品,他们没有得到 FDA 的批准,用于预防、治疗或治愈任何医疗状况、疾病或疾病。我们必须强调,将这些产品以任何形式引入人类或动物的身体都是法律严格禁止的。遵守这些指南对确保研究和实验的法律和道德标准的符合性至关重要。