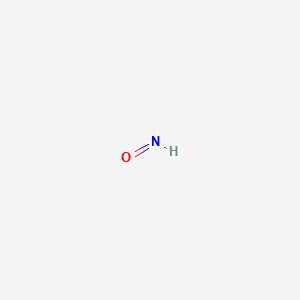
亚硝基
- 点击 快速询问 获取最新报价。
- 提供有竞争力价格的高质量产品,您可以更专注于研究。
描述
Nitroxyl, also known as HNO, is a highly reactive molecule that has gained significant attention in the scientific community due to its potential therapeutic applications. Unlike its closely related counterpart, nitric oxide (NO), which is well known for its vasodilatory effects, nitroxyl has been shown to possess unique pharmacological properties that make it a promising candidate for the treatment of various cardiovascular diseases, cancer, and neurodegenerative disorders.
科学研究应用
化学和生物效应
亚硝酰(HNO)以其独特的化学性质和反应性而闻名,特别是与调节性巯基蛋白的相互作用。这些相互作用对于理解HNO的生物效应至关重要,包括其作为心力衰竭治疗药物的潜力 (Bianco et al., 2017)。
在聚合物稳定中的作用
对“稳定”自由基的研究,包括亚硝基自由基(亚硝基),已经显著扩展。这些自由基已在聚合物的光稳定剂背景下进行了研究,有助于发展更有效的稳定技术 (Scott, 1980)。
治疗潜力和信号传导
HNO作为一氧化氮(NO)的一电子还原形式,具有独特的化学和生物特性。它被探索作为一种血管扩张剂和正肌力药物,可能有助于治疗心力衰竭。它与巯基和含巯基蛋白的相互作用对其生物活性至关重要 (Shoman & Aly, 2015)。
血管张力调节
HNO正逐渐被认为是血管张力的调节因子。研究已经调查了其在阻力动脉内皮依赖性松弛和去极化中的作用,突显了其在血管健康中的潜在重要性 (Andrews等,2009)。
生物系统中的成像
成像技术的创新,如发展线粒体靶向的近红外荧光探针,已经实现了对活细胞中亚硝酰的更精确可视化。这有助于更深入地了解其在生理或病理作用中的作用 (Gong et al., 2016); (Ren et al., 2017)。
实验挑战
在生物系统中研究HNO需要对其独特的化学性质有深刻的理解,以便有效设计和解释实验。这对于探索其新颖的生物活性和药理潜力至关重要 (Fukuto et al., 2008)。
生物应用的受控释放
开发光可控HNO释放化合物对于生物医学研究具有重要意义,可以在细胞研究中实现特定部位和时间控制的HNO释放 (Nakagawa, 2013)。
心血管和中枢神经系统
最近的研究突出了HNO在心血管和中枢神经系统中的影响,强调了其作为心血管疾病治疗药物的潜力 (Kemp-Harper, 2011)。
生物亚硝酰的成像
近红外荧光探针的设计和合成推动了生物亚硝酰的成像,使得可以在活细胞中研究其生物合成和生物活性 (Tan et al., 2015)。
属性
CAS 编号 |
14332-28-6 |
---|---|
产品名称 |
Nitroxyl |
分子式 |
HNO |
分子量 |
31.014 g/mol |
IUPAC 名称 |
nitroxyl |
InChI |
InChI=1S/HNO/c1-2/h1H |
InChI 键 |
ODUCDPQEXGNKDN-UHFFFAOYSA-N |
SMILES |
N=O |
规范 SMILES |
N=O |
其他 CAS 编号 |
14332-28-6 |
同义词 |
azanone HNO compound nitrosyl hydride nitroxide nitroxide radical nitroxyl |
产品来源 |
United States |
体外研究产品的免责声明和信息
请注意,BenchChem 上展示的所有文章和产品信息仅供信息参考。 BenchChem 上可购买的产品专为体外研究设计,这些研究在生物体外进行。体外研究,源自拉丁语 "in glass",涉及在受控实验室环境中使用细胞或组织进行的实验。重要的是要注意,这些产品没有被归类为药物或药品,他们没有得到 FDA 的批准,用于预防、治疗或治愈任何医疗状况、疾病或疾病。我们必须强调,将这些产品以任何形式引入人类或动物的身体都是法律严格禁止的。遵守这些指南对确保研究和实验的法律和道德标准的符合性至关重要。