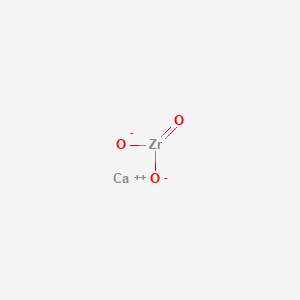
Calcium zirconate
描述
Calcium zirconate (CaZrO₃) is a perovskite-type ceramic material characterized by its orthorhombic crystal structure (space group Pnma) and high melting point (>2,300°C) . It is synthesized via methods such as sol-gel, solid-solid fusion, template-assisted routes, and micro-emulsion, with calcination temperatures typically ranging from 900°C to 1,500°C . Key properties include:
- Thermal Stability: Retains structural integrity up to 1,600°C, making it suitable for refractory applications in metallurgy and aerospace .
- Mechanical Properties: High fracture toughness (2.5–3.0 MPa·m¹/²) and flexural strength (120–150 MPa), comparable to yttria-stabilized zirconia (YSZ) .
- Electrical Conductivity: Pure stoichiometric CaZrO₃ exhibits low oxygen ion conductivity, but non-stoichiometric compositions (e.g., Ca₁₋ₓZrO₃₋δ) show enhanced ionic conductivity due to defect formation .
- Functional Applications: Used in thermal barrier coatings (TBCs), solid oxide fuel cell electrolytes, CO₂ adsorbents, and dielectric ceramics .
准备方法
Mechanochemical Activation-Assisted Solid-State Synthesis
Procedure and Reaction Mechanism
Mechanochemical synthesis involves high-energy ball milling of stoichiometric mixtures of zirconia (ZrO₂) and calcium carbonate (CaCO₃) to induce mechanochemical activation. Planetary ball mills, such as the AGO-2 system, generate intense shear forces that reduce particle size and create defect-rich surfaces, thereby lowering the activation energy for subsequent solid-state reactions . The process occurs in two stages:
-
Mechanical Activation : Milling at 600–800 RPM for 2–4 hours produces a homogenized mixture with CaCO₃ smeared onto ZrO₂ particles, increasing interfacial contact areas by over 70% compared to unmilled precursors .
-
Thermal Annealing : The milled powder is annealed at 950°C for 2–5 hours, where CaCO₃ decomposes to CaO (ΔH = 178 kJ/mol), which reacts with ZrO₂ to form CaZrO₃ via the exothermic reaction:
Critical Parameters and Outcomes
Table 1: Optimization of mechanochemical synthesis parameters
Parameter | Optimal Range | Effect on Product |
---|---|---|
Milling Time | 2–4 hours | Reduces particle size to 50–100 nm |
Annealing Temperature | 950°C | Yields monophasic CaZrO₃ |
Ca:Zr Molar Ratio | 1:1 | Prevents residual CaO/ZrO₂ phases |
Heating Rate | 5°C/min | Minimizes thermal stress cracking |
Prolonged milling (>6 hours) induces amorphization, requiring higher annealing temperatures (>1000°C) to crystallize CaZrO₃. Phase-pure nanocrystalline powders (crystallite size: 20–30 nm) are achievable with this method, though agglomeration remains a challenge .
Conventional Solid-State Reaction
Process Overview
The solid-state reaction between CaCO₃ and ZrO₂ at elevated temperatures is a widely adopted industrial method. Unlike mechanochemical routes, this approach omits milling and relies solely on thermal diffusion:
3 \xrightarrow{800-1000^\circ \text{C}} \text{CaO} + \text{CO}2 \uparrow \quad \text{(Endothermic)}
2 \xrightarrow{1200-1600^\circ \text{C}} \text{CaZrO}3 \quad
Phase Evolution and Sintering
Table 2: Temperature-dependent phase formation in solid-state synthesis
Temperature Range | Phase Composition | Porosity (%) |
---|---|---|
1200°C | CaZrO₃ + cubic ZrO₂ (Ca-stabilized) | 25–30 |
1400°C | CaZrO₃ + minor CaO | 15–20 |
1600°C | Monophasic CaZrO₃ | <10 |
Equimolar Ca:Zr ratios are critical; excess CaO hydrates to Ca(OH)₂ upon air exposure, causing volumetric expansion and sample disintegration . Sintering at 1600°C for 4–6 hours achieves 94–96% theoretical density, but grain growth (>3 µm) limits mechanical strength in refractory applications .
Oxalate Co-Precipitation Route
Synthesis Protocol
This solution-based method involves co-precipitating Ca²⁺ and Zr⁴⁺ ions as oxalate complexes, followed by calcination. Key steps include:
-
Precursor Preparation : Calcium nitrate (Ca(NO₃)₂·4H₂O) and zirconium n-butoxide (Zr(OBuⁿ)₄) are dissolved in n-propanol with acetylacetone (acac) as a stabilizer .
-
Co-Precipitation : Oxalic acid (H₂C₂O₄) is added to the metal solution under shear mixing, forming a mixed metal oxalate precursor:
-
Calcination : The precursor is calcined at 635–900°C to decompose oxalates into CaZrO₃:
Advantages and Limitations
-
Particle Size : Sub-100 nm powders with narrow size distribution (PDI < 0.2) .
-
Sintering : Densification at 1375°C (vs. 1500°C for solid-state), achieving 98% density with dielectric constant ε = 30 and loss tangent tan δ = 0.0004 at 1 kHz .
-
Challenges : Residual carbonate phases form if drying is incomplete, necessitating strict anhydrous conditions during filtration .
Solution Combustion Synthesis
Fuel-Mediated Exothermic Reaction
Urea (CH₄N₂O) serves as a fuel in this redox-based method, where stoichiometric Ca(NO₃)₂·4H₂O and ZrO(NO₃)₂ are dissolved in water and ignited at 400°C . The reaction proceeds as:
4\text{N}2\text{O} \xrightarrow{400^\circ \text{C}} \text{CaZrO}3 + \text{H}2\text{O} \uparrow + \text{CO}2 \uparrow + \text{NO}x \uparrow
Fuel-to-Oxidizer Ratio Optimization
Table 3: Effect of urea concentration on combustion product
Urea (wt%) | Crystallite Size (nm) | Phase Purity (%) | Agglomeration |
---|---|---|---|
2 | 35 ± 8 | 89 | Severe |
6 | 28 ± 5 | 94 | Moderate |
10 | 45 ± 10 | 97 | Mild |
Excess urea (10 wt%) increases flame temperature, promoting crystallite growth but reducing surface area. Calcination at 800°C for 2 hours yields phase-pure CaZrO₃ with 50–60 nm particles .
Hydrothermal Synthesis
Low-Temperature Crystallization
Hydrothermal methods utilize KOH as a mineralizer to facilitate CaZrO₃ nucleation at 200°C under autogenous pressure. Starting materials include Ca(OH)₂ and ZrOCl₂, which react via:
2 + \text{ZrOCl}2 + 2\text{KOH} \rightarrow \text{CaZrO}3 + 2\text{KCl} + 2\text{H}2\text{O}
Influence of Reaction Parameters
-
Ca:Zr Ratio : A 2:1 molar ratio suppresses ZrO₂ impurities, achieving 99% phase purity .
-
Temperature and Time : Crystallite size increases from 30 nm (200°C, 6 hours) to 80 nm (250°C, 12 hours) .
-
Calcination : Post-hydrothermal annealing at 900°C eliminates residual hydroxyl groups, enhancing crystallinity without grain growth .
Comparative Analysis of Synthesis Methods
Table 4: Critical comparison of CaZrO₃ preparation routes
Method | Temperature Range (°C) | Particle Size (nm) | Phase Purity (%) | Relative Cost |
---|---|---|---|---|
Mechanochemical | 950–1000 | 20–50 | 95–98 | Moderate |
Solid-State | 1200–1600 | 1000–3000 | 90–95 | Low |
Oxalate Co-Precipitation | 635–900 | 50–100 | 98–99 | High |
Combustion | 400–800 | 28–60 | 94–97 | Low |
Hydrothermal | 200–900 | 30–80 | 99 | High |
化学反应分析
Solid-State Reaction (Patent Method)
The industrial-scale synthesis involves reacting zirconium ore (ZrO₂·SiO₂) with calcium oxide:
Key Conditions :
-
Temperature: 2600–3600°F (1427–1982°C)
-
Fluxes (e.g., fluorspar) reduce reaction temperatures to ~2900°F (1593°C) .
-
Post-reaction, dicalcium silicate (2CaO·SiO₂) forms a friable slag, enabling mechanical separation of CaZrO₃ crystals (>20 µm) via shaking tables or air classifiers .
CO₂ Adsorption and Carbonation
This compound exhibits reversible CO₂ adsorption at elevated temperatures, forming mixed carbonates:
Adsorption Performance
Sample Composition | Surface Area (m²/g) | CO₂ Adsorption at 600°C (wt%) |
---|---|---|
CaO | 9.37 | 6.88 |
ZrO₂ | 81.97 | 8.39 |
CaZrO₃ | <5 | 7.12 |
Notes :
-
Low surface area of CaZrO₃ (≤5 m²/g) does not hinder CO₂ uptake due to bulk diffusion-driven reactions .
-
Cyclic adsorption-desorption regenerates CaZrO₃, confirmed by XRD phase analysis .
Acid Resistance and Purification
This compound is insoluble in dilute mineral acids, enabling its separation from silicates and impurities:
In contrast, dicalcium silicate reacts readily:
Purification Protocol :
-
Slurry the silicate-zirconate mixture in water (1:10 ratio).
-
Add 10% excess HCl to dissolve silicates.
High-Temperature Stability and Phase Behavior
At temperatures exceeding 1600°C, CaZrO₃ remains stable but may form secondary phases under non-stoichiometric conditions:
科学研究应用
Biomedical Applications
Bone Graft Materials : Calcium zirconate has been explored as a potential bone graft substitute due to its biocompatibility and ability to bond with bone tissue. Research indicates that it promotes osteoconductivity, making it suitable for orthopedic implants and dental applications .
Dental Applications : In dentistry, this compound is utilized in root canal sealers and as a material for endodontic treatments. Its bioactivity supports the regeneration of dental tissues and enhances the sealing properties of root canal systems .
Energy Applications
Solid Oxide Fuel Cells (SOFCs) : CaZrO₃ is employed as an electrolyte material in solid oxide fuel cells due to its high oxygen ion conductivity and thermal stability at elevated temperatures. This application is critical for improving the efficiency and performance of fuel cells .
Catalysts : The compound serves as a catalyst in various chemical processes, including hydrogen production and ammonia synthesis. Its stability under harsh conditions makes it an ideal candidate for catalytic applications in energy conversion technologies .
Ceramic Industry
High-Temperature Ceramics : this compound is used in the production of high-temperature ceramics due to its excellent thermal stability and resistance to chemical corrosion. It is particularly useful in manufacturing refractory materials that can withstand extreme conditions .
Thermal Barrier Coatings (TBCs) : The compound is applied in thermal barrier coatings for gas turbines and diesel engines, helping to reduce operating temperatures and extend the lifespan of components exposed to high heat .
Electroceramics
This compound's dielectric properties make it suitable for various electroceramic applications, including capacitors, resonators, and dielectric resonators. Research has demonstrated its reliability as a dielectric material at elevated temperatures, making it valuable for electronic components that operate under demanding conditions .
Advanced Materials
Composite Materials : this compound has been incorporated into composite materials to enhance mechanical properties and bioactivity. For instance, studies have shown that combining CaZrO₃ with hydroxyapatite can improve densification and mechanical strength in bioceramics used for bone repair .
Nanomaterials : Recent advancements have led to the synthesis of this compound nanoparticles using co-precipitation methods, which exhibit unique properties suitable for various industrial applications . The nanoscale form enhances surface area and reactivity, making it beneficial for catalytic processes.
Data Table: Summary of Applications
Application Area | Specific Use Cases | Key Properties |
---|---|---|
Biomedical | Bone grafts, dental sealers | Biocompatibility, osteoconductivity |
Energy | SOFC electrolytes, catalysts | High conductivity, stability |
Ceramic Industry | High-temperature ceramics, TBCs | Thermal stability, corrosion resistance |
Electroceramics | Capacitors, resonators | Dielectric properties |
Advanced Materials | Composites with hydroxyapatite | Enhanced mechanical properties |
Case Studies
- Orthopedic Implants : A study on this compound-based orthopedic implants demonstrated significant improvements in mechanical strength and bioactivity compared to traditional materials like titanium alloys. The eutectic composition of this compound and calcium-stabilized zirconia showed promising results in enhancing implant integration with bone tissue .
- Fuel Cell Efficiency : Research highlighted the use of this compound in solid oxide fuel cells where it significantly improved the ionic conductivity at high temperatures (up to 800°C), leading to higher energy conversion efficiency compared to conventional electrolyte materials .
作用机制
The mechanism by which calcium zirconate exerts its effects is primarily related to its ionic and electronic conductivity. At low oxygen pressure, it conducts oxygen ions, while at high pressure, it acts as a mixed ionic and electronic conductor . This dual conductivity is crucial for its applications in oxygen sensors and solid oxide fuel cells. The molecular targets and pathways involved include the diffusion of oxygen ions and the interaction with dopants to modify its electrical properties .
相似化合物的比较
Yttria-Stabilized Zirconia (YSZ)
Key Insight : YSZ is widely used in TBCs but is outperformed by CaZrO₃ in high-temperature stability and thermal shock resistance. However, YSZ has superior ionic conductivity in fuel cell applications .
Calcium Titanate (CaTiO₃)
Key Insight : CaTiO₃ has superior dielectric properties but lower thermal stability, limiting its use in extreme environments compared to CaZrO₃ .
Magnesium Aluminate Spinel (MgAl₂O₄)
Property | This compound | MgAl₂O₄ | Reference |
---|---|---|---|
Thermal Conductivity | 2.1–2.5 W/m·K | 3.0–3.5 W/m·K | |
Thermal Shock Resistance | High | Moderate | |
Application | Refractories, TBCs | Transparent armor, catalysts |
Key Insight : MgAl₂O₄ offers higher thermal conductivity but lacks the CO₂ adsorption capability and dielectric stability of CaZrO₃ .
Barium this compound Titanate (BCZT)
Key Insight : BCZT excels in dielectric performance but requires polymer composites for flexibility, whereas CaZrO₃ is inherently rigid and thermally robust .
Data Tables
Table 1: Thermal and Mechanical Properties
Compound | Thermal Conductivity (W/m·K) | Thermal Expansion (×10⁻⁶/K) | Fracture Toughness (MPa·m¹/²) |
---|---|---|---|
This compound | 2.1–2.5 | 8.5–9.0 | 2.5–3.0 |
YSZ | 2.2–3.3 | 10.5–11.0 | 3.0–4.0 |
MgAl₂O₄ | 3.0–3.5 | 7.0–8.0 | 1.5–2.0 |
Table 2: CO₂ Adsorption Performance at 600°C
Material | Adsorption Capacity (wt%) | Preparation Method | Reference |
---|---|---|---|
This compound | 24.84 | Micro-emulsion | |
Calcium Oxide (CaO) | 6.88 | Calcined calcium nitrate | |
Zirconium Oxide (ZrO₂) | 8.39 | Commercial powder |
Note: CaZrO₃ outperforms pure oxides due to synergistic carbonate formation pathways .
Critical Analysis of Conflicting Evidence
- Thermal Resistance : While CaZrO₃ with B₂O₃ additives shows poor thermal shock resistance due to high linear expansion , pure CaZrO₃ exhibits excellent stability in titanium casting molds . This discrepancy highlights the sensitivity of properties to compositional modifications.
- Electrical Conductivity : Stoichiometric CaZrO₃ has low ionic conductivity, but excess CaO or ZrO₂ introduces oxygen vacancies, enhancing conductivity by 2–3 orders of magnitude .
生物活性
Calcium zirconate (CaZrO₃) is a ceramic material that has garnered attention in biomedical applications, particularly for its potential use in bone repair and as a biocompatible implant material. This article explores the biological activity of this compound, emphasizing its properties, mechanisms of action, and relevant case studies.
Overview of this compound
This compound is a perovskite-type oxide with a chemical formula CaZrO₃. It exhibits unique properties such as high thermal stability, good mechanical strength, and bioactivity, making it suitable for various applications in medicine and materials science. Its ability to promote osteogenesis and osseointegration is particularly noteworthy in the context of orthopedic implants.
- Bioactivity : this compound has been shown to induce the formation of hydroxyapatite (HA) when in contact with simulated body fluid (SBF), which is essential for bone integration. The bioactivity is attributed to the release of calcium ions that facilitate the nucleation and growth of HA on its surface .
- Mechanical Strength : Studies indicate that this compound exhibits superior mechanical properties compared to traditional bioceramics such as alumina or zirconia. This includes enhanced toughness and resistance to thermal shock, which are critical for load-bearing applications in the body .
- Biocompatibility : In vivo studies have demonstrated that this compound does not elicit significant inflammatory responses when implanted in biological tissues. Histological evaluations show good integration with surrounding bone tissue, characterized by minimal fibrous encapsulation .
Case Study 1: Osteogenic Response in Rabbit Models
A study involving the implantation of this compound-based materials in New Zealand White rabbits assessed the biological response over a period of 12 weeks. The results indicated significant new bone formation around the implants, with histological analysis revealing active osteoblast proliferation and mineralization. Immunohistochemical staining for collagen type I confirmed the presence of newly formed bone matrix .
Case Study 2: Coatings for Improved Bioactivity
Research has focused on enhancing the bioactivity of this compound through surface modifications. A composite material combining this compound with tricalcium phosphate (TCP) was developed and tested for its ability to form HA layers in SBF. The results showed that the composite exhibited improved bioactive properties compared to pure this compound, indicating a synergistic effect that enhances osteoconductivity .
Data Tables
Property | This compound | Traditional Bioceramics |
---|---|---|
Mechanical Strength (MPa) | 300-500 | 200-400 |
Toughness (MPa·m^0.5) | 10-15 | 5-10 |
Bioactivity (HA Formation Time) | 1-2 weeks | 3-4 weeks |
Inflammatory Response | Minimal | Moderate |
常见问题
Basic Research Questions
Q. What synthesis methods are effective for producing phase-pure calcium zirconate, and how do precursor choices influence the perovskite formation mechanism?
- Methodological Answer: Phase-pure CaZrO₃ can be synthesized via solid-state reactions (e.g., calcining stoichiometric CaO and ZrO₂ at 1300–1500°C) or wet-chemical methods like sol-gel and co-precipitation . Precursor selection impacts reaction kinetics and phase purity. For instance, using zirconium oxychloride and calcium nitrate in co-precipitation requires precise pH control to avoid secondary phases . In fused CaZrO₃ production, arc-melting ensures homogeneity but may introduce impurities if precursors are non-stoichiometric .
Q. What characterization techniques are critical for assessing the structural and thermal properties of this compound?
- Methodological Answer: X-ray diffraction (XRD) is essential to confirm orthorhombic perovskite structure and detect secondary phases (e.g., ZrO₂ or CaO) . Scanning electron microscopy (SEM) reveals grain morphology and porosity, critical for applications like refractories or coatings . Differential scanning calorimetry (DSC) and laser flash analysis measure thermal stability (up to 1600°C) and conductivity, key for thermal barrier coatings (TBCs) .
Q. How can researchers prevent unwanted this compound formation during hydroxyapatite (HA) coating processes on biomedical implants?
- Methodological Answer: Avoid sintering temperatures above 1000°C, as HA decomposes into tricalcium phosphate (TCP), releasing CaO that reacts with ZrO₂ to form CaZrO₃. Rapid thermal processing (<400°C) preserves HA crystallinity, while XRD monitoring ensures phase purity .
Advanced Research Questions
Q. How does iron doping influence the crystal structure and stability of this compound, and what methods validate dopant incorporation?
- Methodological Answer: Fe³⁺ substitutes Zr⁴⁺ in CaZrO₃, inducing lattice distortion and reducing unit cell volume. Sintering at 1350–1500°C increases Fe³⁺ incorporation (8–20 mol%), validated via XRD lattice parameter shifts and energy-dispersive X-ray spectroscopy (EDS) . Extended X-ray absorption fine structure (EXAFS) can further clarify Fe coordination environments.
Q. What experimental approaches optimize this compound’s CO₂ capture efficiency at high temperatures?
- Methodological Answer: Optimize CO₂ partial pressure (2.5 atm) and temperature (650°C) in fixed-bed reactors, achieving ~13.85 wt% adsorption . In-situ XRD tracks carbonate phase formation, while thermogravimetric analysis (TGA) quantifies adsorption capacity. Surface alkalinity, measured via acid-base titration, correlates with CO₂ affinity.
Q. In designing CaZrO₃-based refractories, how do compositional variations (e.g., MgO addition) affect phase stability and corrosion resistance?
- Methodological Answer: Adding MgO promotes CaZrO₃ → Ca₀.₁₅Zr₀.₈₅O₁.₈₅ transformation, enhancing corrosion resistance in cement kilns. Phase evolution is monitored via XRD, while SEM-EDS analyzes interfacial reactions with molten clinker. Corrosion tests at 1555°C reveal liquid-phase formation kinetics .
Q. How does this compound interact with titanium alloys during investment casting, and what methods mitigate decomposition reactions?
- Methodological Answer: At >1600°C, CaZrO₃ decomposes into CaO and ZrO₂, which dissolve into molten Ti-6Al-4V. Mitigation strategies include inert argon atmospheres and controlled cooling rates. Post-casting SEM-EDS identifies reaction zones, while thermodynamic modeling predicts decomposition thresholds .
Q. What are the challenges in using this compound as a thermal barrier coating (TBC), and how are its thermo-physical properties evaluated against YSZ?
- Methodological Answer: CaZrO₃’s lower thermal conductivity (~1.5 W/m·K vs. YSZ’s ~2.5 W/m·K) is advantageous, but sintering above 1300°C causes phase instability. Laser flash analysis measures thermal diffusivity, while thermal cycling tests (up to 1200°C) assess spallation resistance. Challenges include matching coefficients of thermal expansion (CTE) with metallic substrates .
Q. Data Contradictions and Resolution
- Synthesis Mechanisms : highlights BCZT perovskite formation variability based on precursors, while emphasizes stoichiometric fusion for purity. Researchers should cross-validate synthesis routes with in-situ XRD to resolve phase discrepancies.
- CO₂ Adsorption : Calcium silicate outperforms CaZrO₃ in CO₂ capture (14.31 wt% vs. 13.85 wt%) at 650°C . However, CaZrO₃’s stability under cyclic adsorption-desorption conditions remains superior, requiring long-term TGA studies.
Q. Methodological Tables
属性
IUPAC Name |
calcium;dioxido(oxo)zirconium | |
---|---|---|
Source | PubChem | |
URL | https://pubchem.ncbi.nlm.nih.gov | |
Description | Data deposited in or computed by PubChem | |
InChI |
InChI=1S/Ca.3O.Zr/q+2;;2*-1; | |
Source | PubChem | |
URL | https://pubchem.ncbi.nlm.nih.gov | |
Description | Data deposited in or computed by PubChem | |
InChI Key |
BJJVDFHQHSBAFC-UHFFFAOYSA-N | |
Source | PubChem | |
URL | https://pubchem.ncbi.nlm.nih.gov | |
Description | Data deposited in or computed by PubChem | |
Canonical SMILES |
[O-][Zr](=O)[O-].[Ca+2] | |
Source | PubChem | |
URL | https://pubchem.ncbi.nlm.nih.gov | |
Description | Data deposited in or computed by PubChem | |
Molecular Formula |
CaO3Zr | |
Source | PubChem | |
URL | https://pubchem.ncbi.nlm.nih.gov | |
Description | Data deposited in or computed by PubChem | |
Molecular Weight |
179.30 g/mol | |
Source | PubChem | |
URL | https://pubchem.ncbi.nlm.nih.gov | |
Description | Data deposited in or computed by PubChem | |
CAS No. |
12013-47-7 | |
Record name | Calcium zirconium oxide (CaZrO3) | |
Source | EPA Chemicals under the TSCA | |
URL | https://www.epa.gov/chemicals-under-tsca | |
Description | EPA Chemicals under the Toxic Substances Control Act (TSCA) collection contains information on chemicals and their regulations under TSCA, including non-confidential content from the TSCA Chemical Substance Inventory and Chemical Data Reporting. | |
体外研究产品的免责声明和信息
请注意,BenchChem 上展示的所有文章和产品信息仅供信息参考。 BenchChem 上可购买的产品专为体外研究设计,这些研究在生物体外进行。体外研究,源自拉丁语 "in glass",涉及在受控实验室环境中使用细胞或组织进行的实验。重要的是要注意,这些产品没有被归类为药物或药品,他们没有得到 FDA 的批准,用于预防、治疗或治愈任何医疗状况、疾病或疾病。我们必须强调,将这些产品以任何形式引入人类或动物的身体都是法律严格禁止的。遵守这些指南对确保研究和实验的法律和道德标准的符合性至关重要。