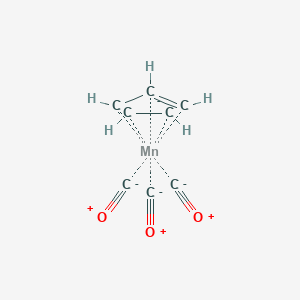
三羰基-π-环戊二烯基锰
概述
描述
Manganese, tricarbonyl-pi-cyclopentadienyl- is an organometallic compound with the chemical formula C₅H₅Mn(CO)₃. It is also known as cyclopentadienylmanganese tricarbonyl. This compound is a member of the metallocene family and is characterized by a manganese atom bonded to a cyclopentadienyl ring and three carbonyl groups. It is commonly used in various chemical reactions and industrial applications due to its unique properties.
科学研究应用
Manganese, tricarbonyl-pi-cyclopentadienyl- has a wide range of applications in scientific research:
Chemistry: It is used as a catalyst in organic synthesis, particularly in reactions involving carbonylation and hydrogenation.
Biology: The compound is studied for its potential use in biological imaging and as a contrast agent in magnetic resonance imaging (MRI).
Medicine: Research is ongoing to explore its potential as an anticancer agent due to its ability to generate reactive oxygen species.
Industry: It is used in the production of fine chemicals and as an additive in fuel to improve combustion efficiency.
作用机制
Target of Action
Manganese, tricarbonyl-pi-cyclopentadienyl-, also known as carbon monoxide;cyclopenta-1,3-diene;manganese, primarily targets the central nervous system and kidneys . It is known to cause changes in these organs upon exposure .
Mode of Action
The compound interacts with its targets through a process of decarbonylation . This is an activation step in which the compound loses a carbonyl group (CO), resulting in the release of carbon monoxide and other toxic or irritating gases . This interaction can lead to changes in the target organs, such as irritation and potential damage .
Biochemical Pathways
It is known that the compound undergoes a rapid reaction with o2 in air , which could potentially affect various oxidative processes within the body
Pharmacokinetics
It is known that the compound can enter the body through inhalation, skin absorption, and ingestion . Once inside the body, it can distribute to various organs, including the central nervous system and kidneys . The compound is also known to sublime at 75-77°C , which could potentially affect its bioavailability.
Result of Action
Exposure to the compound can result in a range of effects, including skin irritation, pulmonary edema, convulsions, and changes in the central nervous system and kidneys . It can also decrease resistance to infection . These effects are likely the result of the compound’s interaction with its targets and the subsequent changes that occur.
Action Environment
The action of the compound can be influenced by various environmental factors. For example, the compound is known to rapidly react with O2 in air , which could potentially affect its stability and efficacy. Additionally, heating the compound can cause it to decompose and produce toxic or irritating gases , which could also influence its action
生化分析
Biochemical Properties
It is known that the compound can interact with various enzymes and proteins, potentially influencing their function
Cellular Effects
It is plausible that the compound could influence cell function by interacting with cellular proteins and enzymes, potentially affecting cell signaling pathways, gene expression, and cellular metabolism .
Molecular Mechanism
It is possible that the compound exerts its effects at the molecular level through binding interactions with biomolecules, potentially leading to enzyme inhibition or activation, and changes in gene expression .
Temporal Effects in Laboratory Settings
It is known that the compound is fairly stable, but can dissociatively chemisorb on surfaces via the loss of one or more carbonyl ligands followed by the oxidative addition of a surface group .
Dosage Effects in Animal Models
The effects of Manganese, tricarbonyl-pi-cyclopentadienyl- at different dosages in animal models have not been extensively studied. It is known that the compound can cause ultrastructural changes in the neurons of rats, leading to altered motor behavior and cognition .
Metabolic Pathways
The metabolic pathways involving Manganese, tricarbonyl-pi-cyclopentadienyl- are not well characterized. Given its potential to interact with various enzymes and proteins, it is plausible that the compound could be involved in various metabolic pathways .
Transport and Distribution
Given its potential to interact with various biomolecules, it is plausible that the compound could be transported and distributed via specific transporters or binding proteins .
Subcellular Localization
The subcellular localization of Manganese, tricarbonyl-pi-cyclopentadienyl- is not well characterized. Given its potential to interact with various biomolecules, it is plausible that the compound could be directed to specific compartments or organelles within the cell .
准备方法
Synthetic Routes and Reaction Conditions
Manganese, tricarbonyl-pi-cyclopentadienyl- can be synthesized through several methods. One common method involves the reaction of manganese pentacarbonyl with cyclopentadiene. The reaction is typically carried out under an inert atmosphere, such as nitrogen or argon, to prevent oxidation. The reaction proceeds as follows:
Mn(CO)5+C5H6→C5H5Mn(CO)3+2CO
The reaction is usually conducted at elevated temperatures, around 150-200°C, and requires a catalyst, such as aluminum chloride, to facilitate the formation of the desired product.
Industrial Production Methods
In industrial settings, the production of manganese, tricarbonyl-pi-cyclopentadienyl- involves large-scale reactors and continuous flow processes. The reaction conditions are optimized to maximize yield and minimize by-products. The use of high-purity starting materials and precise control of reaction parameters, such as temperature and pressure, are crucial for efficient production.
化学反应分析
Types of Reactions
Manganese, tricarbonyl-pi-cyclopentadienyl- undergoes various chemical reactions, including:
Oxidation: The compound can be oxidized to form manganese dioxide and carbon monoxide.
Reduction: It can be reduced to form manganese metal and cyclopentadiene.
Substitution: The carbonyl groups can be substituted with other ligands, such as phosphines or amines.
Common Reagents and Conditions
Oxidation: Common oxidizing agents include oxygen, hydrogen peroxide, and potassium permanganate. The reactions are typically carried out at room temperature or slightly elevated temperatures.
Reduction: Reducing agents such as lithium aluminum hydride or sodium borohydride are used. The reactions are usually conducted in anhydrous solvents under an inert atmosphere.
Substitution: Ligand substitution reactions often require the use of coordinating solvents, such as tetrahydrofuran or dichloromethane, and are carried out at low temperatures to prevent decomposition.
Major Products Formed
Oxidation: Manganese dioxide and carbon monoxide.
Reduction: Manganese metal and cyclopentadiene.
Substitution: Various substituted manganese complexes, depending on the ligands used.
相似化合物的比较
Similar Compounds
Methylcyclopentadienyl manganese tricarbonyl: Similar structure but with a methyl group attached to the cyclopentadienyl ring.
Cyclopentadienylmolybdenum tricarbonyl: Contains molybdenum instead of manganese.
Cyclopentadienylcobalt dicarbonyl: Contains cobalt and has two carbonyl groups instead of three.
Uniqueness
Manganese, tricarbonyl-pi-cyclopentadienyl- is unique due to its specific combination of manganese and cyclopentadienyl ligands, which confer distinct reactivity and stability. Its ability to undergo various chemical reactions and its applications in diverse fields make it a valuable compound in both research and industry.
属性
IUPAC Name |
carbon monoxide;cyclopenta-1,3-diene;manganese | |
---|---|---|
Source | PubChem | |
URL | https://pubchem.ncbi.nlm.nih.gov | |
Description | Data deposited in or computed by PubChem | |
InChI |
InChI=1S/C5H5.3CO.Mn/c1-2-4-5-3-1;3*1-2;/h1-5H;;;;/q-1;;;; | |
Source | PubChem | |
URL | https://pubchem.ncbi.nlm.nih.gov | |
Description | Data deposited in or computed by PubChem | |
InChI Key |
CENDTHIEZAWVHS-UHFFFAOYSA-N | |
Source | PubChem | |
URL | https://pubchem.ncbi.nlm.nih.gov | |
Description | Data deposited in or computed by PubChem | |
Canonical SMILES |
[C-]#[O+].[C-]#[O+].[C-]#[O+].[CH-]1C=CC=C1.[Mn] | |
Source | PubChem | |
URL | https://pubchem.ncbi.nlm.nih.gov | |
Description | Data deposited in or computed by PubChem | |
Molecular Formula |
C8H5MnO3- | |
Source | PubChem | |
URL | https://pubchem.ncbi.nlm.nih.gov | |
Description | Data deposited in or computed by PubChem | |
Molecular Weight |
204.06 g/mol | |
Source | PubChem | |
URL | https://pubchem.ncbi.nlm.nih.gov | |
Description | Data deposited in or computed by PubChem | |
CAS No. |
12079-65-1 | |
Record name | Manganese, tricarbonyl(.eta.5-2,4-cyclopentadien-1-yl)- | |
Source | EPA Chemicals under the TSCA | |
URL | https://www.epa.gov/chemicals-under-tsca | |
Description | EPA Chemicals under the Toxic Substances Control Act (TSCA) collection contains information on chemicals and their regulations under TSCA, including non-confidential content from the TSCA Chemical Substance Inventory and Chemical Data Reporting. | |
Synthesis routes and methods
Procedure details
Retrosynthesis Analysis
AI-Powered Synthesis Planning: Our tool employs the Template_relevance Pistachio, Template_relevance Bkms_metabolic, Template_relevance Pistachio_ringbreaker, Template_relevance Reaxys, Template_relevance Reaxys_biocatalysis model, leveraging a vast database of chemical reactions to predict feasible synthetic routes.
One-Step Synthesis Focus: Specifically designed for one-step synthesis, it provides concise and direct routes for your target compounds, streamlining the synthesis process.
Accurate Predictions: Utilizing the extensive PISTACHIO, BKMS_METABOLIC, PISTACHIO_RINGBREAKER, REAXYS, REAXYS_BIOCATALYSIS database, our tool offers high-accuracy predictions, reflecting the latest in chemical research and data.
Strategy Settings
Precursor scoring | Relevance Heuristic |
---|---|
Min. plausibility | 0.01 |
Model | Template_relevance |
Template Set | Pistachio/Bkms_metabolic/Pistachio_ringbreaker/Reaxys/Reaxys_biocatalysis |
Top-N result to add to graph | 6 |
Feasible Synthetic Routes
体外研究产品的免责声明和信息
请注意,BenchChem 上展示的所有文章和产品信息仅供信息参考。 BenchChem 上可购买的产品专为体外研究设计,这些研究在生物体外进行。体外研究,源自拉丁语 "in glass",涉及在受控实验室环境中使用细胞或组织进行的实验。重要的是要注意,这些产品没有被归类为药物或药品,他们没有得到 FDA 的批准,用于预防、治疗或治愈任何医疗状况、疾病或疾病。我们必须强调,将这些产品以任何形式引入人类或动物的身体都是法律严格禁止的。遵守这些指南对确保研究和实验的法律和道德标准的符合性至关重要。