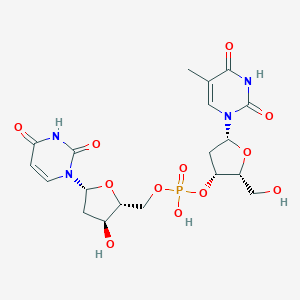
dTpdU
- 点击 快速询问 获取最新报价。
- 提供有竞争力价格的高质量产品,您可以更专注于研究。
描述
dTpdU is a complex organic compound that belongs to the class of nucleoside phosphates. These compounds are essential in various biological processes, including the formation of nucleic acids and energy transfer.
准备方法
Synthetic Routes and Reaction Conditions
The synthesis of dTpdU typically involves the following steps:
Formation of the Nucleoside Base: The pyrimidine bases are synthesized through a series of reactions involving condensation and cyclization.
Glycosylation: The nucleoside base is attached to a sugar moiety (oxolane ring) through glycosylation reactions.
Phosphorylation: The nucleoside is phosphorylated using reagents such as phosphoric acid or phosphoryl chloride under controlled conditions.
Industrial Production Methods
Industrial production of this compound may involve large-scale synthesis using automated reactors and continuous flow processes to ensure high yield and purity. The reaction conditions are optimized to minimize by-products and maximize efficiency.
化学反应分析
Types of Reactions
Oxidation: The compound can undergo oxidation reactions, particularly at the hydroxyl groups.
Reduction: Reduction reactions may occur at the carbonyl groups in the pyrimidine rings.
Substitution: Nucleophilic substitution reactions can take place at the phosphate group.
Common Reagents and Conditions
Oxidizing Agents: Potassium permanganate, hydrogen peroxide.
Reducing Agents: Sodium borohydride, lithium aluminum hydride.
Nucleophiles: Ammonia, amines.
Major Products
The major products formed from these reactions include oxidized or reduced derivatives of the original compound, as well as substituted nucleoside phosphates.
科学研究应用
Chemistry
Synthesis of Nucleic Acids: Used as building blocks in the synthesis of DNA and RNA.
Catalysis: Acts as a catalyst in certain biochemical reactions.
Biology
Cellular Metabolism: Plays a role in energy transfer within cells.
Signal Transduction: Involved in cellular signaling pathways.
Medicine
Antiviral Agents: Used in the development of antiviral drugs.
Cancer Therapy: Investigated for its potential in cancer treatment.
Industry
Biotechnology: Utilized in various biotechnological applications, including genetic engineering and molecular biology research.
作用机制
The compound exerts its effects by interacting with specific molecular targets, such as enzymes and receptors. It participates in biochemical pathways by acting as a substrate or inhibitor, thereby influencing cellular processes.
相似化合物的比较
Similar Compounds
Adenosine Triphosphate (ATP): A key energy carrier in cells.
Cytidine Monophosphate (CMP): A nucleotide involved in RNA synthesis.
Uniqueness
dTpdU is unique due to its specific structure, which allows it to participate in specialized biochemical reactions and pathways.
属性
CAS 编号 |
10318-59-9 |
---|---|
分子式 |
C19H25N4O12P |
分子量 |
532.4 g/mol |
IUPAC 名称 |
[(2R,3S,5R)-5-(2,4-dioxopyrimidin-1-yl)-3-hydroxyoxolan-2-yl]methyl [(2R,3R,5R)-2-(hydroxymethyl)-5-(5-methyl-2,4-dioxopyrimidin-1-yl)oxolan-3-yl] hydrogen phosphate |
InChI |
InChI=1S/C19H25N4O12P/c1-9-6-23(19(29)21-17(9)27)16-5-11(12(7-24)33-16)35-36(30,31)32-8-13-10(25)4-15(34-13)22-3-2-14(26)20-18(22)28/h2-3,6,10-13,15-16,24-25H,4-5,7-8H2,1H3,(H,30,31)(H,20,26,28)(H,21,27,29)/t10-,11+,12+,13+,15+,16+/m0/s1 |
InChI 键 |
JEFGXHFUMBYQPS-PCKPYIHYSA-N |
SMILES |
CC1=CN(C(=O)NC1=O)C2CC(C(O2)CO)OP(=O)(O)OCC3C(CC(O3)N4C=CC(=O)NC4=O)O |
手性 SMILES |
CC1=CN(C(=O)NC1=O)[C@H]2C[C@H]([C@H](O2)CO)OP(=O)(O)OC[C@@H]3[C@H](C[C@@H](O3)N4C=CC(=O)NC4=O)O |
规范 SMILES |
CC1=CN(C(=O)NC1=O)C2CC(C(O2)CO)OP(=O)(O)OCC3C(CC(O3)N4C=CC(=O)NC4=O)O |
同义词 |
2'-deoxyuridylyl-(5'-3')thymidine dTpdU thymidylyl-(3'-5')deoxyuridine |
产品来源 |
United States |
体外研究产品的免责声明和信息
请注意,BenchChem 上展示的所有文章和产品信息仅供信息参考。 BenchChem 上可购买的产品专为体外研究设计,这些研究在生物体外进行。体外研究,源自拉丁语 "in glass",涉及在受控实验室环境中使用细胞或组织进行的实验。重要的是要注意,这些产品没有被归类为药物或药品,他们没有得到 FDA 的批准,用于预防、治疗或治愈任何医疗状况、疾病或疾病。我们必须强调,将这些产品以任何形式引入人类或动物的身体都是法律严格禁止的。遵守这些指南对确保研究和实验的法律和道德标准的符合性至关重要。