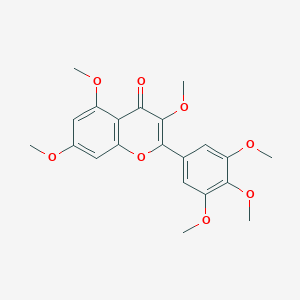
六甲氧基杨梅素
描述
Molecular Structure Analysis
The molecular structure of Hexa-O-methylmyricitin includes 21 carbon atoms, 22 hydrogen atoms, and 8 oxygen atoms . It has a total of 53 bonds, including 31 non-H bonds, 14 multiple bonds, 7 rotatable bonds, 2 double bonds, 12 aromatic bonds, 3 six-membered rings, 1 ten-membered ring, 1 aromatic ketone, 1 aliphatic ether, and 6 aromatic ethers .Physical And Chemical Properties Analysis
The physical and chemical properties of Hexa-O-methylmyricitin include a molecular formula of C21H22O8 and an average mass of 402.395 Da . It contains a total of 53 bonds, including 31 non-H bonds, 14 multiple bonds, 7 rotatable bonds, 2 double bonds, 12 aromatic bonds, 3 six-membered rings, 1 ten-membered ring, 1 aromatic ketone, 1 aliphatic ether, and 6 aromatic ethers .科学研究应用
己烯糖酸的生色团: Rosenau 等人(2017 年)讨论了在制浆条件下己烯糖酸 (HexA) 的形成及其在纸浆中产生颜色的作用。他们合成了 HexA 模型单元并研究了它们的主要降解中间体,提供了对特定条件下相关化合物化学行为的见解 (Rosenau 等人,2017).
二十六烷醇和神经再生: Azzouz 等人(1996 年)探讨了正二十六烷醇 (hexa) 对神经再生的影响。他们的研究表明,hexa 在提高小鼠坐骨神经损伤后感觉纤维的再生率和改善功能恢复方面具有潜力 (Azzouz 等人,1996).
重组 β-己糖胺酶 A 的生产: Akeboshi 等人(2007 年)对重组人 β-己糖胺酶 A (HexA) 的生产进行了研究,这对于治疗 GM2 神经节苷脂沉积症非常重要。这项研究为酶替代疗法和生物技术生产方法提供了有价值的信息 (Akeboshi 等人,2007).
己醛和食品安全: Lanciotti 等人(2003 年)研究了己醛和相关化合物对食品应用中致病菌的抗菌活性。他们的研究结果表明,这些化合物在提高最低限度加工食品的卫生安全方面具有潜在用途 (Lanciotti 等人,2003).
自组装有机单分子层和蛋白质吸附: Prime 和 Whitesides(1991 年)使用自组装单分子层 (SAMs) 研究了蛋白质与有机表面的相互作用,包括具有六(乙二醇)末端的 SAM。这项研究与理解蛋白质在各种表面上的吸附行为有关 (Prime 和 Whitesides,1991).
未来方向
The future directions for research on Hexa-O-methylmyricitin could include further investigation into its synthesis, chemical reactions, mechanism of action, and physical and chemical properties. Additionally, research could explore its potential applications in various fields, such as medicine or environmental science .
属性
IUPAC Name |
3,5,7-trimethoxy-2-(3,4,5-trimethoxyphenyl)chromen-4-one | |
---|---|---|
Source | PubChem | |
URL | https://pubchem.ncbi.nlm.nih.gov | |
Description | Data deposited in or computed by PubChem | |
InChI |
InChI=1S/C21H22O8/c1-23-12-9-13(24-2)17-14(10-12)29-19(21(28-6)18(17)22)11-7-15(25-3)20(27-5)16(8-11)26-4/h7-10H,1-6H3 | |
Source | PubChem | |
URL | https://pubchem.ncbi.nlm.nih.gov | |
Description | Data deposited in or computed by PubChem | |
InChI Key |
CMRBCUQYNLDSKE-UHFFFAOYSA-N | |
Source | PubChem | |
URL | https://pubchem.ncbi.nlm.nih.gov | |
Description | Data deposited in or computed by PubChem | |
Canonical SMILES |
COC1=CC2=C(C(=C1)OC)C(=O)C(=C(O2)C3=CC(=C(C(=C3)OC)OC)OC)OC | |
Source | PubChem | |
URL | https://pubchem.ncbi.nlm.nih.gov | |
Description | Data deposited in or computed by PubChem | |
Molecular Formula |
C21H22O8 | |
Source | PubChem | |
URL | https://pubchem.ncbi.nlm.nih.gov | |
Description | Data deposited in or computed by PubChem | |
Molecular Weight |
402.4 g/mol | |
Source | PubChem | |
URL | https://pubchem.ncbi.nlm.nih.gov | |
Description | Data deposited in or computed by PubChem | |
Product Name |
Myricetin hexamethyl ether | |
CAS RN |
14813-27-5 | |
Record name | Hexa-O-methylmyricitin | |
Source | ChemIDplus | |
URL | https://pubchem.ncbi.nlm.nih.gov/substance/?source=chemidplus&sourceid=0014813275 | |
Description | ChemIDplus is a free, web search system that provides access to the structure and nomenclature authority files used for the identification of chemical substances cited in National Library of Medicine (NLM) databases, including the TOXNET system. | |
Record name | HEXA-O-METHYLMYRICITIN | |
Source | FDA Global Substance Registration System (GSRS) | |
URL | https://gsrs.ncats.nih.gov/ginas/app/beta/substances/M5J17BI02D | |
Description | The FDA Global Substance Registration System (GSRS) enables the efficient and accurate exchange of information on what substances are in regulated products. Instead of relying on names, which vary across regulatory domains, countries, and regions, the GSRS knowledge base makes it possible for substances to be defined by standardized, scientific descriptions. | |
Explanation | Unless otherwise noted, the contents of the FDA website (www.fda.gov), both text and graphics, are not copyrighted. They are in the public domain and may be republished, reprinted and otherwise used freely by anyone without the need to obtain permission from FDA. Credit to the U.S. Food and Drug Administration as the source is appreciated but not required. | |
Retrosynthesis Analysis
AI-Powered Synthesis Planning: Our tool employs the Template_relevance Pistachio, Template_relevance Bkms_metabolic, Template_relevance Pistachio_ringbreaker, Template_relevance Reaxys, Template_relevance Reaxys_biocatalysis model, leveraging a vast database of chemical reactions to predict feasible synthetic routes.
One-Step Synthesis Focus: Specifically designed for one-step synthesis, it provides concise and direct routes for your target compounds, streamlining the synthesis process.
Accurate Predictions: Utilizing the extensive PISTACHIO, BKMS_METABOLIC, PISTACHIO_RINGBREAKER, REAXYS, REAXYS_BIOCATALYSIS database, our tool offers high-accuracy predictions, reflecting the latest in chemical research and data.
Strategy Settings
Precursor scoring | Relevance Heuristic |
---|---|
Min. plausibility | 0.01 |
Model | Template_relevance |
Template Set | Pistachio/Bkms_metabolic/Pistachio_ringbreaker/Reaxys/Reaxys_biocatalysis |
Top-N result to add to graph | 6 |
Feasible Synthetic Routes
体外研究产品的免责声明和信息
请注意,BenchChem 上展示的所有文章和产品信息仅供信息参考。 BenchChem 上可购买的产品专为体外研究设计,这些研究在生物体外进行。体外研究,源自拉丁语 "in glass",涉及在受控实验室环境中使用细胞或组织进行的实验。重要的是要注意,这些产品没有被归类为药物或药品,他们没有得到 FDA 的批准,用于预防、治疗或治愈任何医疗状况、疾病或疾病。我们必须强调,将这些产品以任何形式引入人类或动物的身体都是法律严格禁止的。遵守这些指南对确保研究和实验的法律和道德标准的符合性至关重要。