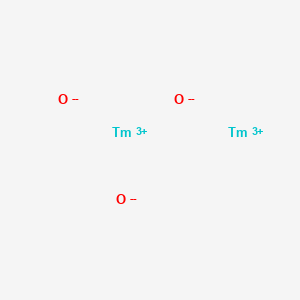
Thulium oxide
描述
Thulium oxide, chemically known as thulium (III) oxide, is a rare earth compound with the formula Tm2O3. It was first isolated in 1879 by Swedish chemist Per Teodor Cleve from an impure sample of erbia. This compound is characterized by its pale green color and cubic crystal structure. It is notable for its unique properties and specialized applications, making it a fascinating compound in the family of rare earth oxides .
Synthetic Routes and Reaction Conditions:
Direct Synthesis from Metal: this compound can be synthesized by directly burning thulium metal in the air. This process requires careful control of the environment to ensure complete conversion to thulium (III) oxide. The reaction proceeds as follows: [ 4 \text{Tm} + 3 \text{O}_2 \rightarrow 2 \text{Tm}_2\text{O}_3 ]
Decomposition of Oxoacid Salts: Another method involves the thermal decomposition of thulium oxoacid salts, such as thulium nitrate.
Industrial Production Methods:
Hydrothermal Method: Thulium (III) oxide can also be produced using a hydrothermal method where thulium (III) acetate is mixed with an ammonia solution, causing thulium (III) oxide to precipitate as a white solid.
Types of Reactions:
Oxidation: this compound can undergo oxidation reactions, where it reacts with oxygen to form higher oxides.
Reduction: It can be reduced to thulium metal using strong reducing agents.
Substitution: this compound can participate in substitution reactions where it reacts with other compounds to form new products.
Common Reagents and Conditions:
Oxidation: Oxygen gas (O2) under controlled conditions.
Reduction: Strong reducing agents such as hydrogen gas (H2) or lithium aluminum hydride (LiAlH4).
Substitution: Various reagents depending on the desired product, such as halogens or other metal oxides.
Major Products Formed:
Oxidation: Higher oxides of thulium.
Reduction: Thulium metal.
Substitution: New compounds formed by replacing oxygen atoms with other elements.
作用机制
Target of Action
Thulium Oxide (Tm2O3) is a pale green solid compound . It doesn’t have a specific biological target due to its inorganic nature. It’s used in various technological applications due to its unique physical and chemical properties .
Mode of Action
This compound doesn’t interact with biological targets in the way organic compounds or drugs do. Instead, its mode of action is primarily physical, based on its unique properties such as high melting point, density, and slight solubility in acids .
Biochemical Pathways
As an inorganic compound, this compound doesn’t participate in biochemical pathways like organic compounds or enzymes do. Its use is mainly in the field of materials science and technology .
Pharmacokinetics
Like other lanthanides, it’s likely poorly absorbed and slowly excreted .
Result of Action
This compound doesn’t have a direct effect on molecular or cellular functions. Its primary effects are observed in its applications in technology, such as in the production of laser materials, X-ray phosphors, and magnetic bubble materials .
Action Environment
The action of this compound is influenced by environmental factors. For instance, it can absorb water and carbon dioxide from the atmosphere . Its stability under extreme temperatures makes it suitable for high-temperature applications .
科学研究应用
Thulium oxide has a wide range of scientific research applications:
相似化合物的比较
Thulium oxide can be compared with other rare earth oxides such as:
- Yttrium oxide (Y2O3)
- Lutetium oxide (Lu2O3)
- Erbium oxide (Er2O3)
- Terbium oxide (Tb2O3)
Uniqueness of this compound:
- Photodynamic Properties: Unlike many other rare earth oxides, this compound can generate reactive oxygen species under near-infrared light, making it particularly useful in medical applications .
- Thermal Stability: this compound has a high melting point (2341°C) and boiling point (3945°C), which makes it suitable for high-temperature applications .
属性
IUPAC Name |
oxygen(2-);thulium(3+) | |
---|---|---|
Source | PubChem | |
URL | https://pubchem.ncbi.nlm.nih.gov | |
Description | Data deposited in or computed by PubChem | |
InChI |
InChI=1S/3O.2Tm/q3*-2;2*+3 | |
Source | PubChem | |
URL | https://pubchem.ncbi.nlm.nih.gov | |
Description | Data deposited in or computed by PubChem | |
InChI Key |
ZIKATJAYWZUJPY-UHFFFAOYSA-N | |
Source | PubChem | |
URL | https://pubchem.ncbi.nlm.nih.gov | |
Description | Data deposited in or computed by PubChem | |
Canonical SMILES |
[O-2].[O-2].[O-2].[Tm+3].[Tm+3] | |
Source | PubChem | |
URL | https://pubchem.ncbi.nlm.nih.gov | |
Description | Data deposited in or computed by PubChem | |
Molecular Formula |
O3Tm2 | |
Source | PubChem | |
URL | https://pubchem.ncbi.nlm.nih.gov | |
Description | Data deposited in or computed by PubChem | |
DSSTOX Substance ID |
DTXSID50894774 | |
Record name | Thulium oxide (Tm2O3) | |
Source | EPA DSSTox | |
URL | https://comptox.epa.gov/dashboard/DTXSID50894774 | |
Description | DSSTox provides a high quality public chemistry resource for supporting improved predictive toxicology. | |
Molecular Weight |
385.867 g/mol | |
Source | PubChem | |
URL | https://pubchem.ncbi.nlm.nih.gov | |
Description | Data deposited in or computed by PubChem | |
CAS No. |
12036-44-1 | |
Record name | Thulium oxide | |
Source | ChemIDplus | |
URL | https://pubchem.ncbi.nlm.nih.gov/substance/?source=chemidplus&sourceid=0012036441 | |
Description | ChemIDplus is a free, web search system that provides access to the structure and nomenclature authority files used for the identification of chemical substances cited in National Library of Medicine (NLM) databases, including the TOXNET system. | |
Record name | Thulium oxide (Tm2O3) | |
Source | EPA Chemicals under the TSCA | |
URL | https://www.epa.gov/chemicals-under-tsca | |
Description | EPA Chemicals under the Toxic Substances Control Act (TSCA) collection contains information on chemicals and their regulations under TSCA, including non-confidential content from the TSCA Chemical Substance Inventory and Chemical Data Reporting. | |
Record name | Thulium oxide (Tm2O3) | |
Source | EPA DSSTox | |
URL | https://comptox.epa.gov/dashboard/DTXSID50894774 | |
Description | DSSTox provides a high quality public chemistry resource for supporting improved predictive toxicology. | |
Record name | THULIUM OXIDE | |
Source | FDA Global Substance Registration System (GSRS) | |
URL | https://gsrs.ncats.nih.gov/ginas/app/beta/substances/DZJ5EE05EB | |
Description | The FDA Global Substance Registration System (GSRS) enables the efficient and accurate exchange of information on what substances are in regulated products. Instead of relying on names, which vary across regulatory domains, countries, and regions, the GSRS knowledge base makes it possible for substances to be defined by standardized, scientific descriptions. | |
Explanation | Unless otherwise noted, the contents of the FDA website (www.fda.gov), both text and graphics, are not copyrighted. They are in the public domain and may be republished, reprinted and otherwise used freely by anyone without the need to obtain permission from FDA. Credit to the U.S. Food and Drug Administration as the source is appreciated but not required. | |
体外研究产品的免责声明和信息
请注意,BenchChem 上展示的所有文章和产品信息仅供信息参考。 BenchChem 上可购买的产品专为体外研究设计,这些研究在生物体外进行。体外研究,源自拉丁语 "in glass",涉及在受控实验室环境中使用细胞或组织进行的实验。重要的是要注意,这些产品没有被归类为药物或药品,他们没有得到 FDA 的批准,用于预防、治疗或治愈任何医疗状况、疾病或疾病。我们必须强调,将这些产品以任何形式引入人类或动物的身体都是法律严格禁止的。遵守这些指南对确保研究和实验的法律和道德标准的符合性至关重要。