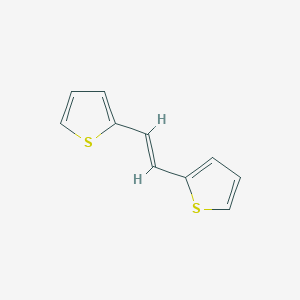
trans-1,2-Di(2-thienyl)ethylene
描述
trans-1,2-Di(2-thienyl)ethylene (trans-DTE) is a conjugated organic compound featuring two thiophene rings connected by a central ethylene double bond (C=C). Its structure (Figure 1) enables extended π-conjugation, making it valuable in materials science, particularly in conductive polymers and organic electronics. Key physical properties include a melting point of 133°C, boiling point of 302.4°C at 760 mmHg, density of 1.268 g/cm³, and a refractive index of 1.728 . Electrochemical studies highlight its utility in forming poly(trans-DTE) films, which exhibit tunable conductivity and sensitivity to overoxidation .
准备方法
Synthetic Routes and Reaction Conditions: The synthesis of trans-1,2-Di(2-thienyl)ethylene typically involves the reaction of thiophene-2-carbaldehyde with titanium tetrachloride and zinc in tetrahydrofuran. The reaction is carried out under controlled temperature conditions, starting at -18°C and gradually warming to room temperature. The mixture is then refluxed for several hours to complete the reaction. The product is isolated by recrystallization from hexane, yielding a yellow solid .
Industrial Production Methods: While specific industrial production methods for this compound are not extensively documented, the general approach involves scaling up the laboratory synthesis procedures. This includes optimizing reaction conditions, using larger reactors, and employing continuous flow techniques to enhance yield and purity.
化学反应分析
Types of Reactions:
Oxidation: trans-1,2-Di(2-thienyl)ethylene can undergo oxidation reactions, typically using oxidizing agents such as hydrogen peroxide or potassium permanganate. These reactions often lead to the formation of sulfoxides or sulfones.
Reduction: Reduction of this compound can be achieved using reducing agents like lithium aluminum hydride, resulting in the formation of dihydro derivatives.
Substitution: The compound can participate in electrophilic substitution reactions, where the thiophene rings are substituted by various electrophiles under acidic conditions.
Common Reagents and Conditions:
Oxidation: Hydrogen peroxide, potassium permanganate.
Reduction: Lithium aluminum hydride.
Substitution: Electrophiles such as halogens, nitrating agents, under acidic conditions.
Major Products Formed:
Oxidation: Sulfoxides, sulfones.
Reduction: Dihydro derivatives.
Substitution: Halogenated or nitrated thiophene derivatives.
科学研究应用
Applications in Organic Electronics
Organic Light-Emitting Diodes (OLEDs)
trans-1,2-Di(2-thienyl)ethylene is utilized as a key material in OLEDs, where it contributes to improved efficiency and brightness. Its ability to facilitate charge transport enhances the overall performance of these devices .
Organic Photovoltaics (OPVs)
In OPVs, this compound plays a crucial role in increasing energy conversion efficiency. Its unique electronic structure allows for effective light absorption and charge separation, making it an essential component in next-generation solar cells .
Conductive Polymers
This compound serves as a fundamental building block in the synthesis of conductive polymers. These polymers are integral to flexible electronic devices due to their mechanical flexibility and excellent electrical conductivity. Research indicates that polymers incorporating this compound exhibit superior thermoelectric properties, making them suitable for energy harvesting applications .
Photonic Applications
The compound's optical properties make it ideal for use in photonic devices such as sensors and lasers. Its ability to manipulate light effectively allows for advancements in optical communication technologies and sensor development .
Material Science Innovations
In material science, this compound is employed to develop advanced materials with tailored electronic and optical characteristics. This includes applications in coatings and adhesives that require enhanced durability and flexibility .
Case Study 1: Thermoelectric Properties
A study investigated the thermoelectric properties of conjugated polymers containing this compound subunits. The results indicated that modifying the backbone structure of these polymers significantly improved their charge transport mobilities and thermoelectric performance when doped with specific agents .
Case Study 2: Synthesis Pathways
Research focusing on organic synthesis has utilized this compound as a model system to explore new synthetic pathways. This work has led to a better understanding of reaction mechanisms involving thiophene derivatives .
Data Table: Summary of Applications
Application Area | Description | Benefits |
---|---|---|
Organic Electronics | Used in OLEDs and OPVs | Enhanced efficiency and performance |
Conductive Polymers | Building block for flexible electronic devices | Excellent conductivity and flexibility |
Photonic Devices | Utilized in sensors and lasers | Effective light manipulation |
Material Science | Development of advanced materials | Tailored electronic and optical properties |
Organic Synthesis | Model system for exploring new synthetic pathways | Insights into reaction mechanisms |
作用机制
The mechanism by which trans-1,2-Di(2-thienyl)ethylene exerts its effects is primarily related to its electronic structure. The conjugated system of the thiophene rings and the ethylene moiety allows for efficient charge transfer and delocalization of electrons. This property is crucial in its role as a semiconductor material. The molecular targets and pathways involved include interactions with other conjugated systems and the formation of charge-transfer complexes.
相似化合物的比较
Comparison with Structurally Similar Compounds
Thiophene-Based Analogues
Poly[trans-1,2-di(2-furyl)ethylene] (DFE)
- Structural Difference : Replaces thiophene with furan rings.
- Key Findings :
- Overoxidation Sensitivity : DFE-based polymers are more prone to overoxidation than poly(trans-DTE), as shown by cyclic voltammetry (CV) .
- Conductivity : Poly(trans-DTE) exhibits electronic conductivity several orders of magnitude higher than DFE .
- Rationale : The electron-rich thiophene stabilizes the polymer backbone better than furan, enhancing charge transport .
1,2-Di(thiophen-2-yl)ethyne
- Structural Difference : Ethyne (C≡C) replaces ethylene (C=C).
- Molecular formula: C₁₀H₆S₂ (vs. C₁₀H₈S₂ for trans-DTE), with a lower molecular weight (190.28 g/mol vs. 192.30 g/mol) .
Aromatic Substituent Variants
trans-1,2-Dibenzoylethylene
- Structural Difference : Benzoyl (C₆H₅CO-) groups replace thiophene.
- Key Properties :
trans-Stilbene (1,2-Diphenylethylene)
- Structural Difference : Benzene replaces thiophene.
- Comparison :
Heteroatom-Functionalized Analogues
trans-1,2-Bis(4-pyridyl)ethylene
- Structural Difference : Pyridyl groups replace thiophene.
- Applications :
Halogenated Derivatives (e.g., trans-1,2-Dichloroethylene)
- Structural Difference : Chlorine substituents replace thiophene.
- Impact : Halogens reduce conjugation and introduce steric hindrance, rendering these compounds less effective in electronic applications compared to trans-DTE .
Performance in Functional Materials
Thermoelectric Materials
- trans-DTE subunits in conjugated polymers (e.g., PEDOT derivatives) show optimized thermoelectric performance via doping engineering, achieving high power factors (e.g., >100 µW/m·K²) .
- Analogues like trans-1,2-bis(4-pyridyl)ethylene lack comparable thermoelectric activity due to poor charge-carrier mobility .
Liquid Crystalline Behavior
- trans-DTE derivatives exhibit enantiotropic nematic phases, whereas stilbene-based vinylenes require longer alkyl chains for similar mesophase stability .
- Example: all-trans-1,2-Bis[5-(4-butylstyryl)-2-thienyl]ethylene displays a nematic phase between 120°C and 150°C, outperforming non-thiophene analogues .
Data Tables
Table 1. Physical Properties of trans-DTE and Analogues
Table 2. Thermal and Phase Behavior
Compound | Mesophase Range (°C) | Phase Type |
---|---|---|
all-trans-1,2-Bis[5-(4-butylstyryl)-2-thienyl]ethylene | 120–150 | Nematic |
trans-Stilbene | >200 (decomposes) | Isotropic |
生物活性
trans-1,2-Di(2-thienyl)ethylene (CAS Number: 13640-78-3) is an organic compound characterized by its unique molecular structure, featuring two thiophene rings attached to an ethylene moiety in a trans configuration. This compound has garnered attention in various fields, particularly due to its potential biological activities, including antimicrobial and anticancer properties. This article delves into the biological activity of this compound, highlighting key research findings, mechanisms of action, and applications.
- Molecular Formula : C10H8S2
- Molecular Weight : 200.36 g/mol
- Density : 1.268 g/cm³
- Melting Point : 133°C
- Boiling Point : 302.4°C at 760 mmHg
These properties contribute to its stability and reactivity in biological systems.
The biological activity of this compound is primarily linked to its interactions with cellular components and biochemical pathways:
- Antimicrobial Activity : The compound exhibits potential antimicrobial effects, possibly through the disruption of microbial cell membranes or interference with metabolic processes.
- Anticancer Properties : Research indicates that derivatives of this compound may induce apoptosis in cancer cells by activating specific signaling pathways or inhibiting cell proliferation.
Antimicrobial Studies
A study investigating the antimicrobial properties of this compound revealed significant activity against various bacterial strains. The compound demonstrated a minimum inhibitory concentration (MIC) that was effective in inhibiting growth in both Gram-positive and Gram-negative bacteria.
Bacterial Strain | MIC (µg/mL) |
---|---|
Staphylococcus aureus | 32 |
Escherichia coli | 64 |
Pseudomonas aeruginosa | 128 |
These results suggest that this compound could be a candidate for developing new antimicrobial agents .
Anticancer Activity
In vitro studies have shown that this compound exhibits selective cytotoxicity towards certain cancer cell lines. For instance, it was found to inhibit the proliferation of breast cancer cells (MCF-7) with an IC50 value of approximately 15 µM. This effect is believed to be mediated through the induction of oxidative stress and subsequent apoptosis .
Cell Line | IC50 (µM) |
---|---|
MCF-7 (Breast Cancer) | 15 |
HeLa (Cervical Cancer) | 20 |
Case Study 1: Antimicrobial Efficacy
A recent publication explored the use of this compound as a lead compound for developing novel antibiotics. The study highlighted its effectiveness against multi-drug resistant strains, suggesting that modifications to its structure could enhance its potency and selectivity .
Case Study 2: Cancer Cell Line Analysis
Another study focused on the anticancer activity of this compound against various cancer cell lines. The findings indicated that combining this compound with established chemotherapeutics resulted in synergistic effects, enhancing overall efficacy while reducing side effects .
常见问题
Basic Research Questions
Q. What are the primary synthetic routes for trans-1,2-Di(2-thienyl)ethylene, and how do reaction conditions influence product purity?
- Methodological Answer : Electrochemical polymerization via anodic oxidation in acetonitrile is a common method, using 2×10⁻³ M solutions of the monomer. Parameters like solvent choice, applied potential, and electrolyte concentration critically affect polymer film quality. For example, acetonitrile reduces side reactions compared to aqueous solutions, improving conductivity . Characterization via cyclic voltammetry and ellipsometry helps monitor film formation and thickness-dependent optical properties .
Q. How are the physical and electronic properties of this compound characterized?
- Methodological Answer : Key properties include melting point (133°C), density (1.268 g/cm³), and refractive index (1.728), measured via differential scanning calorimetry (DSC) and spectroscopic ellipsometry. Electronic properties like HOMO-LUMO gaps are analyzed using UV-Vis spectroscopy and cyclic voltammetry. The compound’s oxidation potential aligns with thiophene derivatives, making it suitable for conductive polymer applications .
Advanced Research Questions
Q. How does structural modulation of this compound enhance thermoelectric performance in conjugated polymers?
- Methodological Answer : Introducing electron-withdrawing/donating groups or doping with iodine improves thermoelectric power factors. For instance, Zhu et al. (2022) optimized thermoelectric performance by tuning side chains and backbone rigidity, achieving higher Seebeck coefficients (≥120 µV/K) and power factors via doping engineering. Stability under thermal stress is assessed using thermogravimetric analysis (TGA) .
Q. What challenges arise from overoxidation in poly[this compound], and how are they mitigated?
- Methodological Answer : Overoxidation degrades conductivity by introducing carbonyl defects. Comparative studies show poly[this compound] (PDTE) is less sensitive than furan analogs (PDFE). Mitigation strategies include:
- Limiting anodic potential during synthesis to avoid overoxidation thresholds.
- Using ionic liquids as electrolytes to stabilize the polymer matrix .
Q. How do DFT and SERS studies resolve conformational ambiguities in this compound derivatives?
- Methodological Answer : Density functional theory (DFT) with B3LYP/6-31++G(d,p) basis sets predicts vibrational modes and molecular polarizability. Surface-enhanced Raman spectroscopy (SERS) on Ag/Au substrates identifies adsorption geometries. For example, Hu et al. (2015) assigned Raman peaks to specific ring vibrations and confirmed planar adsorption via angle-resolved spectra .
Q. What experimental designs enable comparative studies between this compound and its analogs (e.g., furyl or pyridyl derivatives)?
- Methodological Answer : Controlled synthesis of analogs (e.g., trans-1,2-Di(2-furyl)ethylene) under identical electrochemical conditions allows direct comparison of conductivity and stability. Ellipsometry and impedance spectroscopy quantify differences in charge carrier mobility, while XPS identifies oxidation-induced defects .
Q. How is the stability of this compound assessed in optoelectronic devices?
- Methodological Answer : Accelerated aging tests under UV irradiation and thermal cycling (e.g., 85°C/85% humidity) evaluate degradation. Photoluminescence quenching and AFM surface morphology analysis correlate stability with device lifetime. PDTE shows superior stability over PDFE in OLED prototypes due to reduced overoxidation .
Q. Key Research Findings
- Thermoelectric Optimization : Doping with iodine increases conductivity (σ ≈ 150 S/cm) while maintaining Seebeck coefficients >100 µV/K .
- Overoxidation Sensitivity : PDTE retains 80% conductivity after 100 redox cycles, outperforming PDFE (50% retention) .
- SERS Applications : DFT-predicted Raman modes align with experimental SERS, confirming planar adsorption on Au substrates .
属性
IUPAC Name |
2-[(E)-2-thiophen-2-ylethenyl]thiophene | |
---|---|---|
Source | PubChem | |
URL | https://pubchem.ncbi.nlm.nih.gov | |
Description | Data deposited in or computed by PubChem | |
InChI |
InChI=1S/C10H8S2/c1-3-9(11-7-1)5-6-10-4-2-8-12-10/h1-8H/b6-5+ | |
Source | PubChem | |
URL | https://pubchem.ncbi.nlm.nih.gov | |
Description | Data deposited in or computed by PubChem | |
InChI Key |
AYBFWHPZXYPJFW-AATRIKPKSA-N | |
Source | PubChem | |
URL | https://pubchem.ncbi.nlm.nih.gov | |
Description | Data deposited in or computed by PubChem | |
Canonical SMILES |
C1=CSC(=C1)C=CC2=CC=CS2 | |
Source | PubChem | |
URL | https://pubchem.ncbi.nlm.nih.gov | |
Description | Data deposited in or computed by PubChem | |
Isomeric SMILES |
C1=CSC(=C1)/C=C/C2=CC=CS2 | |
Source | PubChem | |
URL | https://pubchem.ncbi.nlm.nih.gov | |
Description | Data deposited in or computed by PubChem | |
Molecular Formula |
C10H8S2 | |
Source | PubChem | |
URL | https://pubchem.ncbi.nlm.nih.gov | |
Description | Data deposited in or computed by PubChem | |
Molecular Weight |
192.3 g/mol | |
Source | PubChem | |
URL | https://pubchem.ncbi.nlm.nih.gov | |
Description | Data deposited in or computed by PubChem | |
Retrosynthesis Analysis
AI-Powered Synthesis Planning: Our tool employs the Template_relevance Pistachio, Template_relevance Bkms_metabolic, Template_relevance Pistachio_ringbreaker, Template_relevance Reaxys, Template_relevance Reaxys_biocatalysis model, leveraging a vast database of chemical reactions to predict feasible synthetic routes.
One-Step Synthesis Focus: Specifically designed for one-step synthesis, it provides concise and direct routes for your target compounds, streamlining the synthesis process.
Accurate Predictions: Utilizing the extensive PISTACHIO, BKMS_METABOLIC, PISTACHIO_RINGBREAKER, REAXYS, REAXYS_BIOCATALYSIS database, our tool offers high-accuracy predictions, reflecting the latest in chemical research and data.
Strategy Settings
Precursor scoring | Relevance Heuristic |
---|---|
Min. plausibility | 0.01 |
Model | Template_relevance |
Template Set | Pistachio/Bkms_metabolic/Pistachio_ringbreaker/Reaxys/Reaxys_biocatalysis |
Top-N result to add to graph | 6 |
Feasible Synthetic Routes
体外研究产品的免责声明和信息
请注意,BenchChem 上展示的所有文章和产品信息仅供信息参考。 BenchChem 上可购买的产品专为体外研究设计,这些研究在生物体外进行。体外研究,源自拉丁语 "in glass",涉及在受控实验室环境中使用细胞或组织进行的实验。重要的是要注意,这些产品没有被归类为药物或药品,他们没有得到 FDA 的批准,用于预防、治疗或治愈任何医疗状况、疾病或疾病。我们必须强调,将这些产品以任何形式引入人类或动物的身体都是法律严格禁止的。遵守这些指南对确保研究和实验的法律和道德标准的符合性至关重要。