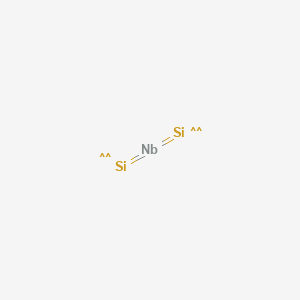
二硅化铌 (NbSi2)
描述
Niobium silicide (NbSi2) is an intermetallic compound composed of niobium and silicon. It is known for its high melting point, excellent high-temperature strength, and good oxidation resistance. These properties make niobium silicide a promising material for high-temperature structural applications, particularly in the aerospace and energy industries .
科学研究应用
Niobium silicide has a wide range of scientific research applications due to its unique properties:
Chemistry: In chemistry, niobium silicide is used as a high-temperature material for catalytic reactions and as a component in advanced composite materials .
Biology: While its applications in biology are limited, niobium silicide’s biocompatibility and corrosion resistance make it a potential candidate for biomedical implants and devices .
Medicine: Niobium silicide’s high-temperature stability and resistance to oxidation make it suitable for use in medical devices that require sterilization and high-temperature processing .
Industry: In the aerospace industry, niobium silicide is used in the production of turbine blades, rocket nozzles, and other high-temperature components. Additionally, niobium silicide is used in the energy industry for high-temperature coatings and components in gas turbines and other power generation systems .
作用机制
Target of Action
Niobium silicide (NbSi₂) primarily targets high-temperature structural applications due to its excellent mechanical properties, such as high hardness, creep resistance, and high melting point . It is used in aerospace and other industries where materials must withstand extreme temperatures and mechanical stress .
Mode of Action
NbSi₂ interacts with its targets by forming a dense, homogeneous coating on niobium substrates. This interaction involves the diffusion of silicon into the niobium substrate, creating a composite layer of NbSi₂ and pure silicon on the surface . The resulting changes include enhanced hardness and resistance to oxidation, which are critical for high-temperature applications .
Biochemical Pathways
While NbSi₂ is not involved in biological systems, its formation and stability are influenced by the chemical pathways during its synthesis. The process involves the deposition of silicon on niobium and subsequent diffusion to form a stable NbSi₂ layer. This pathway ensures the material’s structural integrity and resistance to environmental degradation .
Pharmacokinetics
In the context of materials science, the pharmacokinetics of NbSi₂ can be likened to its processing and performance characteristics. The absorption (A) involves the diffusion of silicon into niobium. Distribution (D) refers to the uniform formation of the NbSi₂ layer. Metabolism (M) can be seen as the chemical reactions during the synthesis process, and excretion (E) would be the removal of any unreacted silicon or by-products. These properties impact the material’s overall performance and durability .
Result of Action
The molecular and cellular effects of NbSi₂’s action include increased hardness, improved oxidation resistance, and enhanced mechanical stability at high temperatures. These effects make NbSi₂ an ideal material for applications requiring robust performance under extreme conditions .
Action Environment
Environmental factors such as temperature, silicon availability, and processing conditions significantly influence NbSi₂’s action, efficacy, and stability. Higher temperatures and optimal silicon availability during synthesis lead to better quality coatings with higher hardness and improved structural integrity . Additionally, the presence of oxygen can affect the oxidation resistance of NbSi₂, making it crucial to control the environment during its application .
Niobium silicide’s unique properties and interactions make it a valuable material for high-temperature applications, ensuring durability and performance in demanding environments.
: Characterization of NbSi₂: Correlation between Crystal Structure and Mechanical Properties : Synthesis of Si-NbSi₂ coatings on Nb substrate by hot dip silicon-plating method
准备方法
Synthetic Routes and Reaction Conditions: Niobium silicide can be synthesized through various methods, including solid-state diffusion, hot dip silicon-plating, and pack cementation. In solid-state diffusion, niobium and silicon powders are mixed and heated to high temperatures (1000-1150°C) to form niobium silicide . Hot dip silicon-plating involves immersing a niobium substrate in molten silicon, resulting in the formation of a niobium silicide coating . Pack cementation involves placing niobium in a powder mixture containing silicon, an activator (such as ammonium fluoride), and an inert filler (such as alumina) and heating it to high temperatures .
Industrial Production Methods: Industrial production of niobium silicide typically involves pack cementation due to its ability to produce uniform coatings and its scalability. This method is widely used to create protective coatings on niobium-based components exposed to high temperatures and aggressive environments .
化学反应分析
Types of Reactions: Niobium silicide undergoes various chemical reactions, including oxidation, reduction, and substitution.
Oxidation: When exposed to high temperatures, niobium silicide forms a protective oxide layer, primarily composed of niobium pentoxide (Nb2O5). the formation of this oxide layer can lead to volume expansion and spalling, limiting its high-temperature applications .
Reduction: Niobium silicide can be reduced using strong reducing agents such as hydrogen or carbon monoxide, resulting in the formation of niobium and silicon .
Substitution: Substitution reactions involve replacing silicon atoms in the niobium silicide lattice with other elements, such as chromium or titanium, to enhance its properties .
Common Reagents and Conditions: Common reagents used in these reactions include oxygen (for oxidation), hydrogen or carbon monoxide (for reduction), and various metal halides (for substitution). These reactions typically occur at high temperatures (above 1000°C) to facilitate the necessary chemical transformations .
Major Products: The major products formed from these reactions include niobium pentoxide (Nb2O5) during oxidation, elemental niobium and silicon during reduction, and modified niobium silicide compounds during substitution .
相似化合物的比较
Niobium silicide can be compared with other similar compounds, such as molybdenum silicide (MoSi2), tungsten silicide (WSi2), and titanium silicide (TiSi2).
Molybdenum Silicide (MoSi2): Molybdenum silicide has a similar high melting point and oxidation resistance but exhibits better electrical conductivity and is often used in heating elements and electronic devices .
Tungsten Silicide (WSi2): Tungsten silicide also shares high-temperature stability and oxidation resistance but has a higher density and is used in applications requiring heavy-duty materials .
Titanium Silicide (TiSi2): Titanium silicide is known for its excellent electrical conductivity and is widely used in microelectronics and semiconductor devices .
Uniqueness of Niobium Silicide: Niobium silicide stands out due to its combination of high melting point, excellent mechanical properties, and good oxidation resistance, making it particularly suitable for high-temperature structural applications in the aerospace and energy industries .
By understanding the unique properties and applications of niobium silicide, researchers and engineers can continue to develop advanced materials and technologies that leverage its strengths.
属性
InChI |
InChI=1S/Nb.2Si | |
---|---|---|
Source | PubChem | |
URL | https://pubchem.ncbi.nlm.nih.gov | |
Description | Data deposited in or computed by PubChem | |
InChI Key |
GXAAOBARRLPQRY-UHFFFAOYSA-N | |
Source | PubChem | |
URL | https://pubchem.ncbi.nlm.nih.gov | |
Description | Data deposited in or computed by PubChem | |
Canonical SMILES |
[Si]=[Nb]=[Si] | |
Source | PubChem | |
URL | https://pubchem.ncbi.nlm.nih.gov | |
Description | Data deposited in or computed by PubChem | |
Molecular Formula |
NbSi2 | |
Source | PubChem | |
URL | https://pubchem.ncbi.nlm.nih.gov | |
Description | Data deposited in or computed by PubChem | |
Molecular Weight |
149.08 g/mol | |
Source | PubChem | |
URL | https://pubchem.ncbi.nlm.nih.gov | |
Description | Data deposited in or computed by PubChem | |
CAS No. |
12034-80-9 | |
Record name | Niobium silicide (NbSi2) | |
Source | CAS Common Chemistry | |
URL | https://commonchemistry.cas.org/detail?cas_rn=12034-80-9 | |
Description | CAS Common Chemistry is an open community resource for accessing chemical information. Nearly 500,000 chemical substances from CAS REGISTRY cover areas of community interest, including common and frequently regulated chemicals, and those relevant to high school and undergraduate chemistry classes. This chemical information, curated by our expert scientists, is provided in alignment with our mission as a division of the American Chemical Society. | |
Explanation | The data from CAS Common Chemistry is provided under a CC-BY-NC 4.0 license, unless otherwise stated. | |
Record name | Niobium silicide (NbSi2) | |
Source | EPA Chemicals under the TSCA | |
URL | https://www.epa.gov/chemicals-under-tsca | |
Description | EPA Chemicals under the Toxic Substances Control Act (TSCA) collection contains information on chemicals and their regulations under TSCA, including non-confidential content from the TSCA Chemical Substance Inventory and Chemical Data Reporting. | |
Record name | Niobium disilicide | |
Source | European Chemicals Agency (ECHA) | |
URL | https://echa.europa.eu/substance-information/-/substanceinfo/100.031.635 | |
Description | The European Chemicals Agency (ECHA) is an agency of the European Union which is the driving force among regulatory authorities in implementing the EU's groundbreaking chemicals legislation for the benefit of human health and the environment as well as for innovation and competitiveness. | |
Explanation | Use of the information, documents and data from the ECHA website is subject to the terms and conditions of this Legal Notice, and subject to other binding limitations provided for under applicable law, the information, documents and data made available on the ECHA website may be reproduced, distributed and/or used, totally or in part, for non-commercial purposes provided that ECHA is acknowledged as the source: "Source: European Chemicals Agency, http://echa.europa.eu/". Such acknowledgement must be included in each copy of the material. ECHA permits and encourages organisations and individuals to create links to the ECHA website under the following cumulative conditions: Links can only be made to webpages that provide a link to the Legal Notice page. | |
体外研究产品的免责声明和信息
请注意,BenchChem 上展示的所有文章和产品信息仅供信息参考。 BenchChem 上可购买的产品专为体外研究设计,这些研究在生物体外进行。体外研究,源自拉丁语 "in glass",涉及在受控实验室环境中使用细胞或组织进行的实验。重要的是要注意,这些产品没有被归类为药物或药品,他们没有得到 FDA 的批准,用于预防、治疗或治愈任何医疗状况、疾病或疾病。我们必须强调,将这些产品以任何形式引入人类或动物的身体都是法律严格禁止的。遵守这些指南对确保研究和实验的法律和道德标准的符合性至关重要。