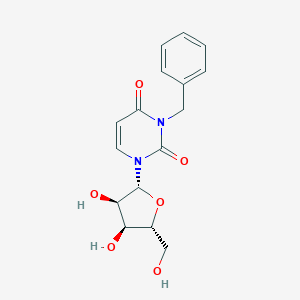
Uridine, 3-(phenylmethyl)-
- 点击 快速询问 获取最新报价。
- 提供有竞争力价格的高质量产品,您可以更专注于研究。
描述
Uridine, 3-(phenylmethyl)-, also known as Uridine, 3-(phenylmethyl)-, is a useful research compound. Its molecular formula is C16H18N2O6 and its molecular weight is 334.32 g/mol. The purity is usually 95%.
The exact mass of the compound Uridine, 3-(phenylmethyl)- is unknown and the complexity rating of the compound is unknown. Its Medical Subject Headings (MeSH) category is Chemicals and Drugs Category - Carbohydrates - Glycosides - Nucleosides - Pyrimidine Nucleosides - Supplementary Records. The storage condition is unknown. Please store according to label instructions upon receipt of goods.
BenchChem offers high-quality Uridine, 3-(phenylmethyl)- suitable for many research applications. Different packaging options are available to accommodate customers' requirements. Please inquire for more information about Uridine, 3-(phenylmethyl)- including the price, delivery time, and more detailed information at info@benchchem.com.
常见问题
Basic Research Questions
Q. What are the established synthetic routes for 3-(phenylmethyl)uridine, and what analytical methods are critical for verifying its purity and structure?
Answer: Synthesis typically involves nucleophilic substitution or benzylation of uridine derivatives. Key steps include protecting the ribose hydroxyl groups to ensure regioselective benzylation at the 3-position. Post-synthesis, purity is assessed via reverse-phase HPLC (C18 column, acetonitrile/water gradient) and thin-layer chromatography (TLC, silica gel) . Structural confirmation requires:
- NMR spectroscopy : 1H- and 13C-NMR to confirm benzyl group integration and uridine backbone integrity.
- Mass spectrometry : High-resolution ESI-MS to verify molecular ion peaks (e.g., [M+H]+ or [M+Na]+) .
- Melting point analysis : Consistency with literature values ensures crystallinity and purity.
Q. How can researchers optimize the solubility and stability of 3-(phenylmethyl)uridine in aqueous and organic solvents for in vitro assays?
Answer:
- Solubility testing : Use DMSO as a stock solvent (10–50 mM) with dilution into phosphate-buffered saline (PBS) or cell culture media. Monitor precipitation via dynamic light scattering (DLS) .
- Stability studies : Employ LC-MS to track degradation under varying pH (4–9), temperatures (4–37°C), and light exposure. Add antioxidants (e.g., 0.1% BHT) to organic solvents to prevent oxidation .
Q. What spectroscopic databases or reference standards are recommended for characterizing 3-(phenylmethyl)uridine derivatives?
Answer:
- PubChem or ChemSpider : Cross-reference NMR and MS data with existing entries for uridine analogs .
- Commercial standards : Use uridine or benzylated nucleoside standards (e.g., Sigma-Aldrich) as chromatographic retention time controls .
Advanced Research Questions
Q. How can contradictory spectroscopic data for 3-(phenylmethyl)uridine derivatives be resolved in structural elucidation?
Answer:
- Multi-technique validation : Combine 1H-1H COSY and HSQC NMR to resolve overlapping proton signals caused by benzyl group rotamers .
- X-ray crystallography : If crystals are obtainable, compare experimental unit cell parameters with Cambridge Structural Database entries .
- Computational modeling : Use DFT calculations (e.g., Gaussian) to simulate NMR chemical shifts and match experimental data .
Q. What experimental designs are suitable for investigating the enzymatic stability of 3-(phenylmethyl)uridine in biological systems?
Answer:
- In vitro enzyme assays : Incubate the compound with nucleoside phosphorylases (e.g., thymidine phosphorylase) and quantify degradation via UV-Vis spectroscopy (λ = 260 nm) .
- Metabolite profiling : Use 14C-labeled 3-(phenylmethyl)uridine and autoradiography to track metabolic byproducts in cell lysates .
- LC-MS/MS : Develop MRM transitions for parent and potential metabolites (e.g., de-benzylated uridine) .
Q. How can researchers address batch-to-batch variability in 3-(phenylmethyl)uridine synthesis for reproducible pharmacological studies?
Answer:
- Process optimization : Standardize reaction conditions (e.g., temperature, solvent ratios) using design-of-experiments (DoE) approaches .
- Quality control (QC) protocols : Implement in-process HPLC monitoring and stringent post-synthesis QC (e.g., ≥95% purity by area normalization) .
- Data sharing : Use FAIR-compliant repositories (e.g., Chemotion) to document synthesis parameters and analytical metadata .
Q. What strategies are effective for studying the cellular uptake and subcellular localization of 3-(phenylmethyl)uridine?
Answer:
- Fluorescent tagging : Conjugate with Cy5 or FITC via a cleavable linker and image via confocal microscopy .
- Radiolabeling : Synthesize 3H-labeled derivatives and quantify uptake via scintillation counting in fractionated organelles .
- Mass spectrometry imaging (MSI) : Use MALDI-TOF to map spatial distribution in tissue sections .
属性
CAS 编号 |
14985-34-3 |
---|---|
分子式 |
C16H18N2O6 |
分子量 |
334.32 g/mol |
IUPAC 名称 |
3-benzyl-1-[(2R,3R,4S,5R)-3,4-dihydroxy-5-(hydroxymethyl)oxolan-2-yl]pyrimidine-2,4-dione |
InChI |
InChI=1S/C16H18N2O6/c19-9-11-13(21)14(22)15(24-11)17-7-6-12(20)18(16(17)23)8-10-4-2-1-3-5-10/h1-7,11,13-15,19,21-22H,8-9H2/t11-,13-,14-,15-/m1/s1 |
InChI 键 |
DBYRVSQCTWOQRS-NMFUWQPSSA-N |
SMILES |
C1=CC=C(C=C1)CN2C(=O)C=CN(C2=O)C3C(C(C(O3)CO)O)O |
手性 SMILES |
C1=CC=C(C=C1)CN2C(=O)C=CN(C2=O)[C@H]3[C@@H]([C@@H]([C@H](O3)CO)O)O |
规范 SMILES |
C1=CC=C(C=C1)CN2C(=O)C=CN(C2=O)C3C(C(C(O3)CO)O)O |
同义词 |
N(3)-benzyluridine |
产品来源 |
United States |
体外研究产品的免责声明和信息
请注意,BenchChem 上展示的所有文章和产品信息仅供信息参考。 BenchChem 上可购买的产品专为体外研究设计,这些研究在生物体外进行。体外研究,源自拉丁语 "in glass",涉及在受控实验室环境中使用细胞或组织进行的实验。重要的是要注意,这些产品没有被归类为药物或药品,他们没有得到 FDA 的批准,用于预防、治疗或治愈任何医疗状况、疾病或疾病。我们必须强调,将这些产品以任何形式引入人类或动物的身体都是法律严格禁止的。遵守这些指南对确保研究和实验的法律和道德标准的符合性至关重要。