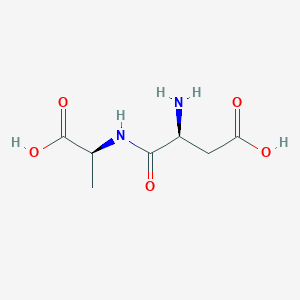
天冬氨酰丙氨酸
概述
描述
This compound is naturally found in the brain and plays a role in enhancing synaptic transmission . It is a part of the broader class of dipeptides, which are organic compounds containing two amino acids joined by a peptide bond.
科学研究应用
Biochemistry
Aspartylalanine serves as a model substrate for studying peptide bond formation and cleavage by enzymes such as peptidases. Understanding these interactions is crucial for drug development and protein research. It can also be used to investigate enzyme kinetics, particularly the activity of β-aspartyl peptidase, which catalyzes the hydrolysis of β-aspartyl peptides.
Neuroscience
In neuroscience, aspartylalanine is studied for its role in synaptic transmission. It has been shown to increase the amplitude of miniature endplate potentials (MEPPs) in phrenic nerve-diaphragm preparations from rats, suggesting its involvement in neurotransmission and neural signaling. This property highlights its potential therapeutic applications in neurological disorders characterized by impaired neurotransmission.
Pharmacology
Research is ongoing to explore the therapeutic potential of aspartylalanine in treating various neurological conditions. Its ability to enhance synaptic transmission positions it as a candidate for developing treatments aimed at improving cognitive functions or alleviating symptoms of neurodegenerative diseases.
Synthetic Applications
Aspartylalanine can be utilized as a building block in the design of synthetic peptides that mimic specific protein-protein interaction motifs essential for cellular signaling. This application underscores its significance in developing novel therapeutic strategies and understanding protein interactions within biological systems.
Material Science
The compound's properties may lead to innovative applications in creating biomaterials for drug delivery systems. Its ability to form hydrogen bonds allows it to self-organize into ordered structures, which can be utilized in designing self-assembling hydrogels.
Metabolomic Profiling
A study on BALB/c macrophages infected with Leishmania demonstrated alterations in amino acid metabolism, including changes associated with L-arginine levels that could be influenced by dipeptides like aspartylalanine. The findings suggest that understanding these interactions may help identify new drug targets for controlling infections.
Synthetic Applications
Recent investigations have explored the use of aspartylalanine in synthetic peptides designed to mimic specific protein interaction motifs crucial for cellular signaling. This application underscores its significance in developing novel therapeutic strategies and understanding protein interactions within biological systems.
作用机制
Target of Action
Aspartylalanine is a dipeptide composed of aspartic acid and alanine . It primarily targets the β-aspartyl peptidase enzyme . This enzyme plays a crucial role in the breakdown of peptide bonds in proteins, specifically those involving aspartic acid residues .
Mode of Action
Aspartylalanine interacts with its target, β-aspartyl peptidase, by serving as a substrate for the enzyme . The enzyme cleaves the peptide bond in aspartylalanine, leading to the release of aspartic acid and alanine . This interaction and the resulting changes play a significant role in protein metabolism .
Biochemical Pathways
Aspartylalanine is involved in the aspartate pathway, which is significant in the synthesis of β-alanine . The aspartate pathway, catalyzed by l-aspartate-α-decarboxylase (ADC), is a critical rate-limiting step in β-alanine synthesis . The downstream effects of this pathway include the production of β-alanine, which is an essential component in various biological circuits .
Pharmacokinetics
Asparaginase exhibits an increase in elimination over time and a marked increase in enzyme activity levels from induction to reinduction
Result of Action
The primary result of aspartylalanine’s action is the breakdown of the peptide into its constituent amino acids, aspartic acid and alanine . This breakdown is crucial for protein metabolism and the regulation of various biochemical pathways .
Action Environment
The action of aspartylalanine is influenced by the aqueous environment in which it is present . The solvation properties of the carboxylate groups in aspartylalanine are significantly influenced by the water molecules in the environment . This interaction can affect the conformation of the dipeptide, potentially influencing its action, efficacy, and stability .
生化分析
Biochemical Properties
Aspartylalanine participates in several biochemical reactions . It interacts with enzymes such as L-asparaginase, which catalyzes the hydrolysis of L-asparagine, a process of high importance in healthcare and food industries .
Cellular Effects
In cellular processes, Aspartylalanine has been found to enhance synaptic transmission. Specifically, it increases the amplitude of the miniature endplate potentials (MEPPs) in phrenic nerve-diaphragm preparations from rats .
Molecular Mechanism
The molecular mechanism of Aspartylalanine involves its interaction with other biomolecules. For instance, it participates in aqueous solvation of carboxylate groups, a process that includes distributed multipole electrostatics and induction contributions .
Metabolic Pathways
Aspartylalanine is involved in the metabolism of asparagine, an important amino acid in mammals. Asparagine acts as a major form of nitrogen storage and transport to sink tissues .
Subcellular Localization
Understanding how it is directed to specific compartments or organelles could provide valuable insights into its role in cellular processes .
准备方法
Synthetic Routes and Reaction Conditions: Aspartylalanine can be synthesized through various methods, including both chemical and enzymatic routes. One common method involves the condensation of aspartic acid with alanine under specific reaction conditions. The reaction typically requires a coupling agent, such as carbodiimide, to facilitate the formation of the peptide bond.
Industrial Production Methods: In industrial settings, the production of aspartylalanine often involves the use of protecting groups to prevent unwanted side reactions. For example, the amino group of aspartic acid can be protected using carbobenzoxy or formyl groups. The protected aspartic acid is then reacted with alanine methyl ester, followed by the removal of the protective groups to yield aspartylalanine .
化学反应分析
Types of Reactions: Aspartylalanine undergoes various chemical reactions, including:
Oxidation: Aspartylalanine can be oxidized to form corresponding oxo derivatives.
Reduction: Reduction reactions can convert aspartylalanine into its reduced forms.
Substitution: Substitution reactions can occur at the amino or carboxyl groups, leading to the formation of different derivatives.
Common Reagents and Conditions:
Oxidation: Common oxidizing agents include hydrogen peroxide and potassium permanganate.
Reduction: Reducing agents such as sodium borohydride are often used.
Substitution: Reagents like acyl chlorides and alkyl halides are used for substitution reactions.
Major Products: The major products formed from these reactions depend on the specific reagents and conditions used. For example, oxidation may yield oxo derivatives, while substitution can produce various substituted dipeptides .
相似化合物的比较
Aspartylalanine is unique among dipeptides due to its specific combination of aspartic acid and alanine. Similar compounds include:
Aspartylphenylalanine: Another dipeptide with different amino acid composition.
Aspartylglutamine: A dipeptide with glutamine instead of alanine.
Aspartylserine: A dipeptide with serine replacing alanine.
These similar compounds differ in their amino acid composition, which affects their chemical properties and biological activities .
生物活性
Aspartylalanine, a dipeptide composed of aspartic acid and alanine, has garnered attention in various fields of biological research due to its unique properties and potential applications. This article explores its biological activity, mechanisms of action, and implications for therapeutic development.
Structure and Properties
Aspartylalanine (CAS Number: 13433-02-8) is classified as a dipeptide, which consists of two amino acids linked by a peptide bond. Its molecular structure allows it to participate in various biochemical processes, particularly in the brain where it is involved in enhancing synaptic transmission . The compound's solubility and interaction with water molecules are critical for its function in biological systems, influencing its behavior within cellular environments.
Biological Functions
1. Role in Synaptic Transmission
Aspartylalanine is naturally present in the brain and plays a significant role in enhancing synaptic transmission. Studies have shown that it increases the amplitude of miniature endplate potentials (MEPPs) in phrenic nerve-diaphragm preparations from rats, indicating its potential involvement in neurotransmission and neural signaling.
2. Interaction with Enzymes
Aspartylalanine serves as a substrate for β-aspartyl peptidase, an enzyme that catalyzes the breakdown of peptides into their constituent amino acids. This enzymatic activity is crucial for various metabolic pathways, including the aspartate pathway, which is significant in synthesizing β-alanine .
3. Potential Therapeutic Applications
Research is ongoing to explore the therapeutic potential of aspartylalanine in neurological disorders. Its ability to enhance synaptic transmission suggests that it may have applications in treating conditions characterized by impaired neurotransmission. Furthermore, its properties as a model compound for peptide synthesis make it valuable in drug development and protein research.
The mechanisms through which aspartylalanine exerts its effects involve several biochemical pathways:
- Peptide Bond Formation : Aspartylalanine can serve as a model substrate for studying peptide bond formation and cleavage by enzymes such as peptidases. Understanding these interactions aids in drug development and protein research.
- Hydrogen Bonding : The compound's ability to form hydrogen bonds allows it to self-organize into ordered structures, which can be utilized in designing self-assembling hydrogels for drug delivery applications.
- Metabolic Pathways : Aspartylalanine participates in the metabolism of asparagine, an essential amino acid involved in nitrogen storage and transport within tissues. This metabolic role highlights its importance in maintaining cellular homeostasis .
Research Findings
Recent studies have provided insights into the biological activity of aspartylalanine:
-
Case Study: Metabolomic Profiling
A study on BALB/c macrophages infected with Leishmania demonstrated alterations in amino acid metabolism, including changes associated with L-arginine levels that could be influenced by dipeptides like aspartylalanine. The findings suggest that understanding these interactions may help identify new drug targets for controlling infections . -
Synthetic Applications
Aspartylalanine has been explored for its potential use in synthetic peptides designed to mimic specific protein-protein interaction motifs crucial for cellular signaling. This application underscores its significance in developing novel therapeutic strategies.
Data Summary
Property | Value |
---|---|
Molecular Formula | C7H12N2O5 |
Molecular Weight | 188.18 g/mol |
Solubility | Highly soluble |
Biological Role | Enhances synaptic transmission |
Enzyme Interaction | β-aspartyl peptidase |
Future Directions
The ongoing research into aspartylalanine's biological activity suggests several promising avenues:
- Neuropharmacology : Further studies are needed to elucidate its precise role in neurotransmission and potential benefits in treating neurological disorders.
- Biomaterials Development : The properties of aspartylalanine may lead to innovative applications in creating biomaterials for drug delivery systems.
- Metabolic Studies : Investigating its interactions within metabolic pathways could provide insights into broader physiological processes and disease mechanisms.
属性
IUPAC Name |
(3S)-3-amino-4-[[(1S)-1-carboxyethyl]amino]-4-oxobutanoic acid | |
---|---|---|
Source | PubChem | |
URL | https://pubchem.ncbi.nlm.nih.gov | |
Description | Data deposited in or computed by PubChem | |
InChI |
InChI=1S/C7H12N2O5/c1-3(7(13)14)9-6(12)4(8)2-5(10)11/h3-4H,2,8H2,1H3,(H,9,12)(H,10,11)(H,13,14)/t3-,4-/m0/s1 | |
Source | PubChem | |
URL | https://pubchem.ncbi.nlm.nih.gov | |
Description | Data deposited in or computed by PubChem | |
InChI Key |
DVUFTQLHHHJEMK-IMJSIDKUSA-N | |
Source | PubChem | |
URL | https://pubchem.ncbi.nlm.nih.gov | |
Description | Data deposited in or computed by PubChem | |
Canonical SMILES |
CC(C(=O)O)NC(=O)C(CC(=O)O)N | |
Source | PubChem | |
URL | https://pubchem.ncbi.nlm.nih.gov | |
Description | Data deposited in or computed by PubChem | |
Isomeric SMILES |
C[C@@H](C(=O)O)NC(=O)[C@H](CC(=O)O)N | |
Source | PubChem | |
URL | https://pubchem.ncbi.nlm.nih.gov | |
Description | Data deposited in or computed by PubChem | |
Molecular Formula |
C7H12N2O5 | |
Source | PubChem | |
URL | https://pubchem.ncbi.nlm.nih.gov | |
Description | Data deposited in or computed by PubChem | |
DSSTOX Substance ID |
DTXSID10928569 | |
Record name | 3-Amino-4-[(1-carboxyethyl)imino]-4-hydroxybutanoic acid | |
Source | EPA DSSTox | |
URL | https://comptox.epa.gov/dashboard/DTXSID10928569 | |
Description | DSSTox provides a high quality public chemistry resource for supporting improved predictive toxicology. | |
Molecular Weight |
204.18 g/mol | |
Source | PubChem | |
URL | https://pubchem.ncbi.nlm.nih.gov | |
Description | Data deposited in or computed by PubChem | |
CAS No. |
13433-02-8 | |
Record name | Aspartylalanine | |
Source | CAS Common Chemistry | |
URL | https://commonchemistry.cas.org/detail?cas_rn=13433-02-8 | |
Description | CAS Common Chemistry is an open community resource for accessing chemical information. Nearly 500,000 chemical substances from CAS REGISTRY cover areas of community interest, including common and frequently regulated chemicals, and those relevant to high school and undergraduate chemistry classes. This chemical information, curated by our expert scientists, is provided in alignment with our mission as a division of the American Chemical Society. | |
Explanation | The data from CAS Common Chemistry is provided under a CC-BY-NC 4.0 license, unless otherwise stated. | |
Record name | alpha-Aspartylalanine | |
Source | ChemIDplus | |
URL | https://pubchem.ncbi.nlm.nih.gov/substance/?source=chemidplus&sourceid=0013433028 | |
Description | ChemIDplus is a free, web search system that provides access to the structure and nomenclature authority files used for the identification of chemical substances cited in National Library of Medicine (NLM) databases, including the TOXNET system. | |
Record name | 3-Amino-4-[(1-carboxyethyl)imino]-4-hydroxybutanoic acid | |
Source | EPA DSSTox | |
URL | https://comptox.epa.gov/dashboard/DTXSID10928569 | |
Description | DSSTox provides a high quality public chemistry resource for supporting improved predictive toxicology. | |
Retrosynthesis Analysis
AI-Powered Synthesis Planning: Our tool employs the Template_relevance Pistachio, Template_relevance Bkms_metabolic, Template_relevance Pistachio_ringbreaker, Template_relevance Reaxys, Template_relevance Reaxys_biocatalysis model, leveraging a vast database of chemical reactions to predict feasible synthetic routes.
One-Step Synthesis Focus: Specifically designed for one-step synthesis, it provides concise and direct routes for your target compounds, streamlining the synthesis process.
Accurate Predictions: Utilizing the extensive PISTACHIO, BKMS_METABOLIC, PISTACHIO_RINGBREAKER, REAXYS, REAXYS_BIOCATALYSIS database, our tool offers high-accuracy predictions, reflecting the latest in chemical research and data.
Strategy Settings
Precursor scoring | Relevance Heuristic |
---|---|
Min. plausibility | 0.01 |
Model | Template_relevance |
Template Set | Pistachio/Bkms_metabolic/Pistachio_ringbreaker/Reaxys/Reaxys_biocatalysis |
Top-N result to add to graph | 6 |
Feasible Synthetic Routes
Q1: What is the significance of studying the solvation properties of aspartylalanine?
A1: Understanding how aspartylalanine interacts with water molecules is crucial for comprehending its behavior in biological systems. The study by Ren and Ponder [] utilized advanced molecular dynamics simulations employing the Amoebapro force field to investigate the aqueous solvation of the carboxylate group in aspartylalanine. This research revealed that Amoebapro provided a more accurate representation of carboxylate solvation compared to traditional force fields, highlighting the importance of sophisticated computational models in studying peptide solvation.
Q2: How does the solvation of aspartylalanine differ from other similar molecules?
A2: The study by Ren and Ponder [] compared the solvation properties of aspartylalanine with those of the glycine zwitterion. Their findings indicated that the inclusion of distributed multipole electrostatics and induction contributions in the Amoebapro force-field led to significant improvements in predicting solvation properties compared to traditional force fields. This emphasizes the unique solvation characteristics of different peptides and the necessity for accurate force fields in computational studies.
Q3: How is aspartylalanine detected and quantified in biological samples like rat brain extracts?
A3: A study by Quack et al. [] described a sensitive method for determining aspartylalanine levels in rat brain extracts. The method involved a pre-column derivatization step using o-phthaldialdehyde/β-mercaptoethanol followed by separation and quantification using reversed-phase liquid chromatography. This technique allowed researchers to identify and quantify aspartylalanine alongside other acidic peptides, showcasing the capability of advanced analytical techniques for analyzing complex biological samples.
A4: The research by Quack et al. [] identified aspartylalanine in rat cerebral cortex at a mean concentration of 4.0 ± 0.4 μM. While the specific role of aspartylalanine in the brain remains unknown, its presence, along with other γ-glutamyl and β-aspartyl peptides, suggests potential involvement in neurotransmission, metabolism, or other physiological processes within the brain. Further research is needed to elucidate the precise biological function of this dipeptide.
体外研究产品的免责声明和信息
请注意,BenchChem 上展示的所有文章和产品信息仅供信息参考。 BenchChem 上可购买的产品专为体外研究设计,这些研究在生物体外进行。体外研究,源自拉丁语 "in glass",涉及在受控实验室环境中使用细胞或组织进行的实验。重要的是要注意,这些产品没有被归类为药物或药品,他们没有得到 FDA 的批准,用于预防、治疗或治愈任何医疗状况、疾病或疾病。我们必须强调,将这些产品以任何形式引入人类或动物的身体都是法律严格禁止的。遵守这些指南对确保研究和实验的法律和道德标准的符合性至关重要。