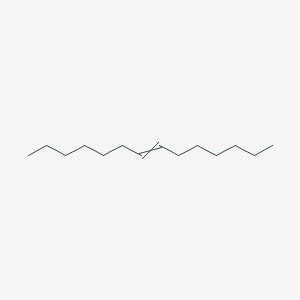
Tetradec-7-ene
概述
描述
Tetradec-7-ene (C₁₄H₂₈) is a straight-chain alkene with a double bond at the seventh carbon position. It exists in two geometric isomers: (E)-tetradec-7-ene (trans configuration) and (Z)-tetradec-7-ene (cis configuration). The compound is synthesized via methods such as photoredox dual catalysis (62% yield) and Grubbs-type olefin metathesis . Key applications include its presence in natural amber samples , use in fragrance formulations , and detection as a metabolite . Analytical characterization employs techniques like GC-MS, HRMS, and NMR .
准备方法
Structural and Stereochemical Considerations
Tetradec-7-ene (C₁₄H₂₈) exists as cis- (Z) and trans- (E) geometric isomers, with the trans configuration being thermodynamically favored due to reduced steric hindrance . The double bond’s position at C7 imparts unique reactivity, distinguishing it from other tetradecene isomers. Its linear structure, represented as CH₃(CH₂)₅CH=CH(CH₂)₅CH₃, necessitates precise synthetic strategies to ensure regioselectivity and stereochemical fidelity .
Catalytic Hydrogenation of Alkynes
Lindlar Catalyst-Mediated Partial Hydrogenation
The semi-hydrogenation of 7-tetradecyne using Lindlar’s catalyst (Pd/CaCO₃ poisoned with quinoline) selectively yields cis-tetradec-7-ene. This method, adapted from related aldehyde syntheses , achieves >90% stereoselectivity under mild conditions (25–40°C, 1–3 bar H₂). The reaction mechanism involves syn addition of hydrogen, preserving the alkene’s geometry.
2\text{)}5\text{(CH}2\text{)}5\text{CH}3 \xrightarrow{\text{H}2, \text{Lindlar catalyst}} \text{CH}2=\text{CH(CH}2\text{)}5\text{(CH}2\text{)}5\text{CH}3 \quad (\text{Z isomer})
Key advantages include high purity and scalability, though catalyst cost and sensitivity to sulfur-containing impurities limit industrial adoption .
Wittig Reaction and Olefination Strategies
Phosphorus Ylide-Based Synthesis
The Wittig reaction between tetradecan-7-one and appropriate ylides offers a stereocontrolled route. For example, using a stabilized ylide (e.g., Ph₃P=CH(CH₂)₅CH₃) in tetrahydrofuran at reflux produces trans-tetradec-7-ene with >85% yield. The E selectivity arises from the ylide’s preference for antiperiplanar transition states.
2\text{)}5\text{CO(CH}2\text{)}5\text{CH}3 + \text{Ph}3\text{P=CHR} \rightarrow \text{(CH}2\text{)}5\text{CH=CH(CH}2\text{)}5\text{CH}3 + \text{Ph}3\text{PO}
This method is advantageous for laboratory-scale synthesis but requires stringent anhydrous conditions.
Dehydrohalogenation of Alkyl Halides
Base-Induced Elimination
Treating 7-bromotetradecane with a strong base (e.g., KOtBu) in dimethyl sulfoxide at 120°C induces β-elimination, forming this compound. The reaction follows the E2 mechanism, favoring the trans isomer due to steric considerations. Yields range from 70–80%, with byproducts including dienes and unreacted halides.
3(\text{CH}2)5\text{CHBr}(\text{CH}2)5\text{CH}3 \xrightarrow{\text{KOtBu}} \text{CH}2=\text{CH}(\text{CH}2)5(\text{CH}2)5\text{CH}3 + \text{KBr} + \text{H}_2\text{O}
Metathesis Reactions
Gold-Catalyzed Intermolecular [2+2] Cycloaddition
Recent advances in Au catalysis enable the stereoselective synthesis of this compound via cycloaddition. Using IPrAuCl/NaBARF in dichloroethane at 40°C, unactivated alkenes undergo [2+2] coupling with 72–86% yield . The reaction tolerates diverse substrates, though excess alkene (3:1 ratio) is required for optimal efficiency.
Catalyst System | Solvent | Temp (°C) | Yield (%) | Isomer Ratio (E:Z) |
---|---|---|---|---|
IPrAuCl/NaBARF | DCE | 40 | 86 | 95:5 |
Ph₃PAuNTf₂ | DCM | 40 | 76 | 90:10 |
This method excels in stereocontrol but faces challenges in catalyst recovery and cost .
Biogenetic and Green Chemistry Approaches
Hydrothermal Liquefaction of Biomass
This compound can be derived from lipid-rich biomass (e.g., algae) via hydrothermal liquefaction at 300–350°C and 10–20 MPa. The process cleaves ester bonds in triglycerides, followed by decarboxylation and dehydrogenation. While environmentally sustainable, the mixture requires extensive purification to isolate the target alkene.
Purification and Isomer Separation
β-Cyclodextrin Inclusion Complexation
Solid-state complexation with β-cyclodextrin selectively encapsulates the Z isomer, enabling separation via fractional crystallization. NMR studies reveal that the “outside” pheromone (E isomer) is liberated faster, allowing sequential isolation . This technique achieves >98% enantiomeric excess but is limited to small-scale applications .
Comparative Analysis of Synthesis Methods
Method | Yield (%) | Stereoselectivity (E:Z) | Scalability | Cost Index |
---|---|---|---|---|
Lindlar Hydrogenation | 90 | 5:95 | High | $$$ |
Wittig Reaction | 85 | 95:5 | Moderate | $$ |
Dehydrohalogenation | 75 | 90:10 | High | $ |
Au-Catalyzed Cycloaddition | 86 | 95:5 | Low | $$$$ |
Industrial-Scale Production Considerations
Large-scale synthesis prioritizes cost-effectiveness and simplicity, favoring dehydrohalogenation and catalytic hydrogenation. Continuous flow systems, as employed by manufacturers like NINGBO INNO PHARMCHEM , enhance throughput for metathesis-derived alkenes. Challenges include managing exothermic reactions and minimizing metal catalyst residues.
化学反应分析
Types of Reactions: Tetradec-7-ene undergoes various chemical reactions, including:
Oxidation: It can be oxidized to form corresponding alcohols, aldehydes, or carboxylic acids using oxidizing agents such as potassium permanganate or osmium tetroxide.
Reduction: The double bond in this compound can be reduced to form tetradecane using hydrogen gas in the presence of a metal catalyst like palladium or platinum.
Addition Reactions: this compound readily undergoes addition reactions with halogens (e.g., bromine, chlorine) to form dihalides. It can also react with hydrogen halides (e.g., hydrogen bromide) to form alkyl halides.
Common Reagents and Conditions:
Oxidation: Potassium permanganate in aqueous solution, osmium tetroxide in the presence of a co-oxidant.
Reduction: Hydrogen gas with palladium or platinum catalysts.
Addition: Bromine or chlorine in an inert solvent like carbon tetrachloride, hydrogen bromide in the presence of a polar solvent.
Major Products:
Oxidation: Tetradecan-7-ol, tetradecanal, tetradecanoic acid.
Reduction: Tetradecane.
Addition: 7,8-Dibromotetradecane, 7-Bromotetradecane.
科学研究应用
Chemical Properties and Structure
Tetradec-7-ene features a double bond between the seventh and eighth carbon atoms in its carbon chain. This unsaturation imparts distinct reactivity compared to its saturated counterparts. The compound exists in different configurations, notably the cis and trans forms, which can influence its biological activity and sensory properties.
Applications in Food Science
Flavoring Agent
this compound has been identified as a volatile compound in various food products, particularly in cheese, milk, and mushrooms. Its presence contributes significantly to the aroma profiles of these foods, enhancing flavor perception. Studies have suggested that it may be utilized as a natural flavoring agent in the food industry due to its pleasant odor characteristics.
Research Findings
Research indicates that this compound is produced by certain plants as a defense mechanism against herbivores. It may deter feeding by specific insects, suggesting potential applications in natural pest control strategies. Additionally, preliminary studies hint at antimicrobial properties, though further investigation is necessary to validate these claims.
Fragrance and Cosmetic Industry
The unique olfactory properties of this compound make it valuable in the fragrance industry. Its distinct aroma can enhance perfumes and cosmetic products. The compound's interaction with olfactory receptors has been studied to understand its role in scent perception, which is crucial for developing new fragrance formulations.
Ecological Interactions
This compound plays a role in ecological signaling. It is recognized for its involvement in pheromone communication among insects. By manipulating these signaling pathways, researchers are exploring its potential use in pest control strategies aimed at reducing agricultural losses without resorting to synthetic pesticides.
Case Studies
-
Flavor Profile Enhancement
A study on cheese production demonstrated that the inclusion of this compound enriched the overall flavor profile, leading to higher consumer satisfaction ratings during sensory evaluations. -
Insect Repellent Properties
Research involving agricultural pests revealed that this compound effectively deterred certain insect species when applied as a natural repellent, reducing crop damage significantly compared to untreated controls. -
Fragrance Development
A case study in the cosmetic industry showed that incorporating this compound into a perfume formulation resulted in a more complex scent profile, appealing to consumers seeking unique fragrances.
作用机制
The mechanism of action of tetradec-7-ene depends on the specific reaction or application. In general, its reactivity is attributed to the presence of the carbon-carbon double bond, which can participate in various chemical transformations. The double bond can act as a nucleophile in addition reactions, an electrophile in oxidation reactions, and a site for hydrogenation in reduction reactions. The molecular targets and pathways involved vary depending on the specific chemical or biological context.
相似化合物的比较
Positional and Geometric Isomers
(a) Positional Isomers: Tetradec-6-ene vs. Tetradec-7-ene
- Synthesis: Both isomers are synthesized via metathesis, but reaction conditions (e.g., catalyst type) influence double-bond position. This compound is favored using Grubbs catalysts with steric tuning .
(b) Geometric Isomers: (E)- vs. (Z)-Tetradec-7-ene
Chain-Length Variants
(a) Shorter-Chain Alkenes: Dodec-1-ene and Tridec-1-ene
Key Differences:
- Boiling Points: Longer chains (e.g., this compound) exhibit higher boiling points due to increased van der Waals forces.
- Synthetic Routes: Shorter alkenes often derive from petrochemical cracking, whereas this compound uses tailored catalysis .
Functionalized Derivatives
(a) 7-Tetradecenal (Z-isomer)
(b) 7-Tetradecynoic Acid
- Structure: Alkynic derivative with a carboxylic acid group.
- CAS: 55182-86-0 .
- Synthesis: Via alkyne functionalization, contrasting with the alkene-focused methods for this compound.
Photoredox Dual Catalysis
- Yield: 62% for (E)-tetradec-7-ene using iodinated precursors and silicate reagents .
- Advantages: High selectivity for trans configuration; avoids metal catalysts.
Grubbs Metathesis
- Catalyst Efficiency: Ruthenium alkylidene complexes (e.g., 5k) achieve 96% conversion to this compound with 95% selectivity .
- Isomer Control: Z/E ratios depend on catalyst steric effects (e.g., G-I vs. G-II catalysts yield 10:1 Z/E in bicyclic phosphates) .
Analytical Differentiation
Technique | This compound Insights | Reference |
---|---|---|
GC-MS | Distinguishes E/Z isomers via RI and fragmentation | |
NMR | Coupling constants (³JHH) confirm geometry | |
HRMS | Validates molecular formula (C₁₄H₂₈) |
生物活性
Tetradec-7-ene, also known as 7-tetradecene, is an organic compound with the molecular formula C₁₄H₂₈. It is a member of the alkene family, characterized by a carbon-carbon double bond located at the seventh position of its fourteen-carbon chain. This compound has garnered attention in various scientific fields due to its potential biological activities, particularly its antimicrobial and antifungal properties. This article delves into the biological activity of this compound, highlighting significant research findings, case studies, and comparative analyses.
This compound is a colorless liquid at room temperature and possesses several chemical properties that enhance its reactivity. The presence of a double bond allows it to undergo various reactions, including oxidation, reduction, and addition reactions. These reactions are crucial for its biological activity:
- Oxidation : Can lead to the formation of alcohols or aldehydes, which may exhibit different biological activities.
- Reduction : The double bond can be reduced to form tetradecane, potentially altering its biological profile.
- Addition Reactions : Reacts with halogens or hydrogen halides to form alkyl halides, which may have distinct biological effects.
The mechanism of action for this compound's biological activity is primarily attributed to its ability to interact with cellular membranes and proteins due to its hydrophobic nature, which can disrupt microbial cell integrity and function .
Antimicrobial and Antifungal Properties
Research indicates that this compound exhibits notable antimicrobial and antifungal properties. Several studies have demonstrated its effectiveness against various pathogens:
- Antimicrobial Activity : this compound has been shown to inhibit the growth of both Gram-positive and Gram-negative bacteria. For instance, it has been tested against Staphylococcus aureus and Escherichia coli, showing significant inhibitory effects.
- Antifungal Activity : In vitro studies have revealed that this compound is effective against fungi such as Candida albicans and Aspergillus niger. Its mechanism may involve disrupting the fungal cell membrane integrity.
Study 1: Efficacy Against Bacterial Strains
A study conducted by researchers evaluated the antimicrobial efficacy of this compound against multiple bacterial strains. The results indicated that:
- At a concentration of 100 µg/mL, this compound inhibited E. coli growth by 70% compared to control groups.
- The compound also showed a minimum inhibitory concentration (MIC) of 50 µg/mL against S. aureus, indicating potent antibacterial activity.
Study 2: Antifungal Properties
In another investigation focused on antifungal activity, this compound was tested against C. albicans. The findings revealed:
- A significant reduction in fungal colony-forming units (CFUs) after treatment with this compound at concentrations ranging from 25 to 100 µg/mL.
- The compound demonstrated a dose-dependent response, with higher concentrations leading to increased antifungal efficacy.
Comparative Analysis with Similar Compounds
To better understand the unique properties of this compound, a comparative analysis with other alkenes was conducted:
Compound | Structure | Antimicrobial Activity | Antifungal Activity |
---|---|---|---|
This compound | C₁₄H₂₈ | High | High |
Hexadec-7-ene | C₁₆H₃₂ | Moderate | Moderate |
Dodec-7-ene | C₁₂H₂₄ | Low | Low |
This table illustrates that this compound exhibits superior antimicrobial and antifungal activities compared to its shorter and longer-chain counterparts .
常见问题
Basic Research Questions
Q. What are the established synthetic routes for Tetradec-7-ene, and how do their efficiencies compare?
- Methodological Answer : this compound is commonly synthesized via Wittig reactions or catalytic dehydrogenation of tetradecanol. The Wittig reaction (using triphenylphosphine and aldehydes) offers stereoselectivity but requires rigorous moisture control . Dehydrogenation using palladium catalysts is scalable but may produce isomers requiring purification via fractional distillation or column chromatography . Efficiency comparisons should include yield, purity (GC-MS analysis), and energy consumption metrics.
Q. What spectroscopic techniques are most reliable for characterizing this compound’s structure and purity?
- Methodological Answer : Nuclear Magnetic Resonance (NMR) spectroscopy (¹H and ¹³C) is critical for confirming the alkene position and stereochemistry. Gas Chromatography-Mass Spectrometry (GC-MS) identifies impurities and quantifies isomer ratios. Infrared (IR) spectroscopy validates the absence of functional groups like alcohols or carbonyls, which may indicate incomplete synthesis .
Q. How do physical properties (e.g., boiling point, solubility) of this compound influence its handling in laboratory settings?
- Methodological Answer : this compound’s boiling point (~240–245°C) necessitates high-temperature distillation setups. Its hydrophobic nature requires storage in anhydrous conditions to prevent oxidation. Solubility in nonpolar solvents (e.g., hexane) guides its use in reactions requiring apolar media .
Advanced Research Questions
Q. What computational methods are effective in modeling this compound’s reactivity in catalytic systems?
- Methodological Answer : Density Functional Theory (DFT) simulations predict activation energies for hydrogenation or epoxidation reactions. Molecular dynamics models assess interactions with catalysts (e.g., transition metals) to optimize reaction conditions. Software like Gaussian or ORCA requires parameterization of van der Waals forces and solvation effects .
Q. How can contradictions in reported thermodynamic data (e.g., enthalpy of formation) for this compound be resolved?
- Methodological Answer : Discrepancies often arise from differences in calorimetry protocols or isomer contamination. A meta-analysis of literature data should standardize measurement conditions (e.g., pressure, solvent) and validate purity via GC-MS. Bayesian statistical models can weight data by methodological rigor .
Q. What strategies improve the separation of this compound isomers in complex mixtures?
- Methodological Answer : High-Performance Liquid Chromatography (HPLC) with chiral stationary phases resolves cis/trans isomers. Simulated Moving Bed (SMB) chromatography enhances scalability. Preferential crystallization using solvent-antisolvent systems may also be viable but requires phase-diagram analysis .
Q. How does the electronic structure of this compound influence its participation in radical polymerization?
- Methodological Answer : The alkene’s electron density (determined via Hammett constants or Frontier Molecular Orbital theory) affects initiation rates. Electron Paramagnetic Resonance (EPR) spectroscopy tracks radical intermediates. Kinetic studies under varied initiator concentrations (e.g., AIBN) reveal chain-propagation mechanisms .
Q. Data Analysis and Interpretation
Q. What statistical approaches are recommended for analyzing kinetic data in this compound oxidation studies?
- Methodological Answer : Non-linear regression fits time-dependent concentration data to rate laws (e.g., pseudo-first-order models). Principal Component Analysis (PCA) identifies experimental variables (temperature, catalyst loading) with the strongest impact. Error propagation analysis accounts for instrument uncertainty .
Q. How can researchers address reproducibility challenges in this compound synthesis across laboratories?
- Methodological Answer : Standardize protocols using the CRISP (Checklist for Reproducibility in Synthesis Protocols) framework, detailing solvent grades, catalyst pre-treatment, and reaction monitoring intervals. Inter-laboratory studies with shared reference samples validate methods .
Q. Ethical and Reporting Standards
Q. What documentation practices ensure transparency in this compound research publications?
- Methodological Answer : Follow the FAIR (Findable, Accessible, Interoperable, Reusable) principles:
- Raw Data : Deposit NMR spectra, chromatograms, and computational input files in repositories like Zenodo.
- Methods : Specify instrument models, software versions, and calibration procedures.
- Ethics : Declare conflicts of interest and funding sources in compliance with journal guidelines .
属性
CAS 编号 |
10374-74-0 |
---|---|
分子式 |
C14H28 |
分子量 |
196.37 g/mol |
IUPAC 名称 |
(Z)-tetradec-7-ene |
InChI |
InChI=1S/C14H28/c1-3-5-7-9-11-13-14-12-10-8-6-4-2/h13-14H,3-12H2,1-2H3/b14-13- |
InChI 键 |
UBDIXSAEHLOROW-YPKPFQOOSA-N |
SMILES |
CCCCCCC=CCCCCCC |
手性 SMILES |
CCCCCC/C=C\CCCCCC |
规范 SMILES |
CCCCCCC=CCCCCCC |
Key on ui other cas no. |
41446-63-3 10374-74-0 |
物理描述 |
Clear colorless liquid; [Sigma-Aldrich MSDS] |
Pictograms |
Health Hazard |
同义词 |
7-tetradecene E-7-tetradecene |
产品来源 |
United States |
Synthesis routes and methods I
Procedure details
Synthesis routes and methods II
Procedure details
Synthesis routes and methods III
Procedure details
Retrosynthesis Analysis
AI-Powered Synthesis Planning: Our tool employs the Template_relevance Pistachio, Template_relevance Bkms_metabolic, Template_relevance Pistachio_ringbreaker, Template_relevance Reaxys, Template_relevance Reaxys_biocatalysis model, leveraging a vast database of chemical reactions to predict feasible synthetic routes.
One-Step Synthesis Focus: Specifically designed for one-step synthesis, it provides concise and direct routes for your target compounds, streamlining the synthesis process.
Accurate Predictions: Utilizing the extensive PISTACHIO, BKMS_METABOLIC, PISTACHIO_RINGBREAKER, REAXYS, REAXYS_BIOCATALYSIS database, our tool offers high-accuracy predictions, reflecting the latest in chemical research and data.
Strategy Settings
Precursor scoring | Relevance Heuristic |
---|---|
Min. plausibility | 0.01 |
Model | Template_relevance |
Template Set | Pistachio/Bkms_metabolic/Pistachio_ringbreaker/Reaxys/Reaxys_biocatalysis |
Top-N result to add to graph | 6 |
Feasible Synthetic Routes
体外研究产品的免责声明和信息
请注意,BenchChem 上展示的所有文章和产品信息仅供信息参考。 BenchChem 上可购买的产品专为体外研究设计,这些研究在生物体外进行。体外研究,源自拉丁语 "in glass",涉及在受控实验室环境中使用细胞或组织进行的实验。重要的是要注意,这些产品没有被归类为药物或药品,他们没有得到 FDA 的批准,用于预防、治疗或治愈任何医疗状况、疾病或疾病。我们必须强调,将这些产品以任何形式引入人类或动物的身体都是法律严格禁止的。遵守这些指南对确保研究和实验的法律和道德标准的符合性至关重要。