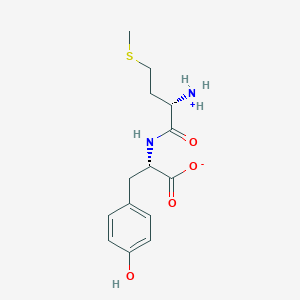
H-MET-TYR-OH
概述
描述
Methionyl-tyrosine is a dipeptide composed of the amino acids methionine and tyrosine. It is represented by the chemical formula C₁₄H₂₀N₂O₄S and has a molar mass of 312.38 g/mol . This compound has been detected in various foods, including birds, chickens, and domestic pigs . Methionyl-tyrosine is expected to form during the digestion of proteins and exists as a zwitterion in aqueous solutions and solid form .
科学研究应用
Methionyl-tyrosine has several scientific research applications:
Chemistry: It is used as a model compound to study peptide bond formation and cleavage reactions.
Biology: Methionyl-tyrosine is studied for its role in protein synthesis and degradation.
作用机制
Target of Action
Methionyl-Tyrosine, also known as H-MET-TYR-OH, primarily targets the enzyme tyrosine hydroxylase . This enzyme plays a crucial role in the synthesis of neurotransmitters and thyroid hormones .
Mode of Action
The compound interacts with its target, tyrosine hydroxylase, by inhibiting its activity . This interaction results in a decrease in the production of neurotransmitters and thyroid hormones .
Biochemical Pathways
Methionyl-Tyrosine affects the biochemical pathway of tyrosine oxidation . Tyrosine, an amino acid, is one of the primary targets for protein oxidation, which plays an essential role in cellular physiology and pathology . The oxidation of tyrosine can generate a diverse range of oxidized products, some of which are important biological intermediates in neurotransmission and eumelanin biosynthesis .
Pharmacokinetics
Factors such as its solubility, stability, and molecular weight (312.39 ) would influence these properties.
Result of Action
The inhibition of tyrosine hydroxylase by Methionyl-Tyrosine can lead to a decrease in the production of neurotransmitters and thyroid hormones . This could potentially affect various physiological processes, including neurotransmission and metabolism .
未来方向
The treatment of chronic myeloid leukemia (CML) has been radically changed by the approval of tyrosine kinase inhibitors (TKIs), which target BCR-ABL1 kinase activity . CML is now managed as a chronic disease requiring long-term treatment and close molecular monitoring . This indicates the potential future directions of Methionyl-Tyrosine in medical treatments.
生化分析
Biochemical Properties
Methionyl-Tyrosine plays a role in various biochemical reactions. Tyrosine, one of the components of Methionyl-Tyrosine, is a proteinogenic amino acid that is essential to many biochemical processes . It is found in the active sites of numerous enzymes and plays an important role in protein–protein and protein-ligand interactions . The phenol functionality in the side chain of Tyrosine allows it to contribute to both hydrophobic interactions through its aromatic ring and hydrogen bonding with binding partners via the hydroxyl group .
Cellular Effects
Methionyl-Tyrosine has been observed to diminish free radical formation in human endothelial cells . This suggests that Methionyl-Tyrosine may have a protective effect on cells, potentially reducing oxidative stress and associated cellular damage.
Molecular Mechanism
The tyrosine component of Methionyl-Tyrosine is known to undergo various post-translational modifications, such as glycosylation, nitration, oxidation, and phosphorylation . These modifications can influence the function of proteins in which Tyrosine is a component, potentially altering cellular processes.
Metabolic Pathways
Tyrosine, a component of Methionyl-Tyrosine, is involved in several metabolic pathways. It is synthesized from prephenate in a process regulated by key enzymes such as phenylalanine hydroxylase and tyrosine aminotransferase . Tyrosine can also be degraded in the liver and to a minor extent in the kidney .
准备方法
Methionyl-tyrosine can be synthesized through peptide bond formation between methionine and tyrosine. One common method involves the use of protecting groups to prevent unwanted side reactions. The synthesis typically involves the following steps:
Protection of Functional Groups: Protecting groups are added to the amino and carboxyl groups of methionine and tyrosine to prevent side reactions.
Activation of Carboxyl Group: The carboxyl group of methionine is activated using reagents such as dicyclohexylcarbodiimide (DCC) or N,N’-diisopropylcarbodiimide (DIC).
Coupling Reaction: The activated methionine is then coupled with the protected tyrosine to form the dipeptide bond.
Deprotection: The protecting groups are removed to yield methionyl-tyrosine.
Industrial production methods may involve solid-phase peptide synthesis (SPPS) or solution-phase synthesis, depending on the scale and desired purity of the product .
化学反应分析
Methionyl-tyrosine undergoes various chemical reactions, including:
Substitution: The amino group of methionine can undergo substitution reactions with electrophiles, leading to the formation of various derivatives.
Common reagents and conditions used in these reactions include cyanogen bromide for cleavage at methionine residues and formic acid for oxidation . Major products formed from these reactions include methionine sulfoxide and various substituted derivatives .
相似化合物的比较
Methionyl-tyrosine can be compared with other dipeptides and amino acid derivatives:
Methionyl-Alanine: Similar to methionyl-tyrosine, methionyl-alanine is a dipeptide composed of methionine and alanine.
Methionyl-Phenylalanine: This dipeptide consists of methionine and phenylalanine.
Tyrosyl-Serine: A dipeptide composed of tyrosine and serine.
Methionyl-tyrosine’s uniqueness lies in its combination of sulfur-containing methionine and aromatic tyrosine, which imparts distinct chemical and biological properties .
属性
IUPAC Name |
(2S)-2-[[(2S)-2-amino-4-methylsulfanylbutanoyl]amino]-3-(4-hydroxyphenyl)propanoic acid | |
---|---|---|
Source | PubChem | |
URL | https://pubchem.ncbi.nlm.nih.gov | |
Description | Data deposited in or computed by PubChem | |
InChI |
InChI=1S/C14H20N2O4S/c1-21-7-6-11(15)13(18)16-12(14(19)20)8-9-2-4-10(17)5-3-9/h2-5,11-12,17H,6-8,15H2,1H3,(H,16,18)(H,19,20)/t11-,12-/m0/s1 | |
Source | PubChem | |
URL | https://pubchem.ncbi.nlm.nih.gov | |
Description | Data deposited in or computed by PubChem | |
InChI Key |
PESQCPHRXOFIPX-RYUDHWBXSA-N | |
Source | PubChem | |
URL | https://pubchem.ncbi.nlm.nih.gov | |
Description | Data deposited in or computed by PubChem | |
Canonical SMILES |
CSCCC(C(=O)NC(CC1=CC=C(C=C1)O)C(=O)O)N | |
Source | PubChem | |
URL | https://pubchem.ncbi.nlm.nih.gov | |
Description | Data deposited in or computed by PubChem | |
Isomeric SMILES |
CSCC[C@@H](C(=O)N[C@@H](CC1=CC=C(C=C1)O)C(=O)O)N | |
Source | PubChem | |
URL | https://pubchem.ncbi.nlm.nih.gov | |
Description | Data deposited in or computed by PubChem | |
Molecular Formula |
C14H20N2O4S | |
Source | PubChem | |
URL | https://pubchem.ncbi.nlm.nih.gov | |
Description | Data deposited in or computed by PubChem | |
DSSTOX Substance ID |
DTXSID10874592 | |
Record name | L-Methionyl-L-tyrosine | |
Source | EPA DSSTox | |
URL | https://comptox.epa.gov/dashboard/DTXSID10874592 | |
Description | DSSTox provides a high quality public chemistry resource for supporting improved predictive toxicology. | |
Molecular Weight |
312.39 g/mol | |
Source | PubChem | |
URL | https://pubchem.ncbi.nlm.nih.gov | |
Description | Data deposited in or computed by PubChem | |
CAS No. |
13589-04-3 | |
Record name | L-Methionyl-L-tyrosine | |
Source | EPA DSSTox | |
URL | https://comptox.epa.gov/dashboard/DTXSID10874592 | |
Description | DSSTox provides a high quality public chemistry resource for supporting improved predictive toxicology. | |
Retrosynthesis Analysis
AI-Powered Synthesis Planning: Our tool employs the Template_relevance Pistachio, Template_relevance Bkms_metabolic, Template_relevance Pistachio_ringbreaker, Template_relevance Reaxys, Template_relevance Reaxys_biocatalysis model, leveraging a vast database of chemical reactions to predict feasible synthetic routes.
One-Step Synthesis Focus: Specifically designed for one-step synthesis, it provides concise and direct routes for your target compounds, streamlining the synthesis process.
Accurate Predictions: Utilizing the extensive PISTACHIO, BKMS_METABOLIC, PISTACHIO_RINGBREAKER, REAXYS, REAXYS_BIOCATALYSIS database, our tool offers high-accuracy predictions, reflecting the latest in chemical research and data.
Strategy Settings
Precursor scoring | Relevance Heuristic |
---|---|
Min. plausibility | 0.01 |
Model | Template_relevance |
Template Set | Pistachio/Bkms_metabolic/Pistachio_ringbreaker/Reaxys/Reaxys_biocatalysis |
Top-N result to add to graph | 6 |
Feasible Synthetic Routes
Q1: What is the molecular formula and weight of Met-Tyr?
A1: The molecular formula of Met-Tyr is C14H18N2O4S, and its molecular weight is 306.37 g/mol.
Q2: What is the structure of Met-Tyr and how does it relate to its activity?
A2: Met-Tyr consists of the amino acids methionine and tyrosine linked by a peptide bond. Studies with Met-Tyr analogues, where specific amino acids are substituted, show the importance of the N-terminal and tyrosine residues for activity. For instance, modifying the lysine at position 6 or the histidine at position 1 in a Met-Tyr-containing dodecapeptide still resulted in significant biological activity. Further research is needed to fully elucidate the structure-activity relationships of Met-Tyr and its derivatives.
Q3: How does Met-Tyr exert its ACE inhibitory effect?
A4: While Met-Tyr is known to inhibit ACE , the exact mechanism is not fully elucidated in the provided research. Further studies are needed to understand whether it acts as a competitive, non-competitive, or uncompetitive inhibitor of ACE.
Q4: How does Met-Tyr contribute to antioxidant defense?
A6: Met-Tyr indirectly protects against oxidative stress by inducing the expression of heme oxygenase-1 (HO-1) and ferritin in human umbilical vein endothelial cells. This induction was found to be independent of its ACE inhibitory activity.
Q5: What is the role of HO-1 in Met-Tyr's antioxidant mechanism?
A7: HO-1, induced by Met-Tyr, catalyzes the degradation of heme into biliverdin, free iron, and carbon monoxide. Bilirubin, the reduced form of biliverdin, exhibits antioxidant properties comparable to those of Met-Tyr in endothelial cell models.
体外研究产品的免责声明和信息
请注意,BenchChem 上展示的所有文章和产品信息仅供信息参考。 BenchChem 上可购买的产品专为体外研究设计,这些研究在生物体外进行。体外研究,源自拉丁语 "in glass",涉及在受控实验室环境中使用细胞或组织进行的实验。重要的是要注意,这些产品没有被归类为药物或药品,他们没有得到 FDA 的批准,用于预防、治疗或治愈任何医疗状况、疾病或疾病。我们必须强调,将这些产品以任何形式引入人类或动物的身体都是法律严格禁止的。遵守这些指南对确保研究和实验的法律和道德标准的符合性至关重要。