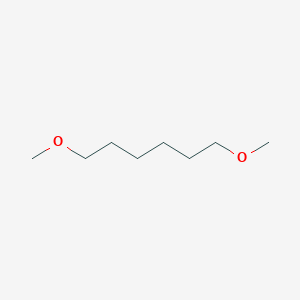
1,6-Dimethoxyhexane
描述
1,6-Dimethoxyhexane is an organic compound with the molecular formula C8H18O2. It is a dialkyl ether, specifically a dimethyl ether of hexane. This compound is characterized by its clear, colorless liquid form and is known for its relatively high boiling point of approximately 182-183°C .
准备方法
Synthetic Routes and Reaction Conditions
1,6-Dimethoxyhexane can be synthesized through the reaction of 1,6-hexanediol with methanol in the presence of an acid catalyst. The reaction typically proceeds under reflux conditions, where the diol undergoes etherification to form the dimethoxy compound .
Industrial Production Methods
In industrial settings, the production of this compound often involves the continuous etherification of 1,6-hexanediol with methanol. This process is optimized for high yield and purity, utilizing advanced catalytic systems and controlled reaction environments to ensure efficient conversion and minimal by-products .
化学反应分析
Types of Reactions
1,6-Dimethoxyhexane primarily undergoes substitution reactions due to the presence of ether linkages. These reactions can include:
Nucleophilic Substitution: The ether groups can be replaced by nucleophiles under appropriate conditions.
Common Reagents and Conditions
Nucleophilic Substitution: Common reagents include halides and other nucleophiles, often in the presence of a base.
Oxidation: Reagents such as potassium permanganate or chromium trioxide can be used under acidic or basic conditions.
Major Products Formed
Substitution Reactions: Products can include various substituted ethers or alcohols.
Oxidation Reactions: Products can include hexanoic acid or hexanal, depending on the extent of oxidation.
科学研究应用
Toxicological Studies
Overview of Toxicity
DMH has been investigated for its toxicological effects, particularly concerning reproductive health. Research indicates that DMH exhibits significant testicular toxicity in male rats, characterized by a reduction in testis weight and degeneration of seminiferous tubules. In a study where male Sprague-Dawley rats were administered varying doses of DMH, significant decreases in testis and thymus weights were observed, alongside alterations in serum biochemical markers such as lactate dehydrogenase and uric acid levels .
Case Study: Testicular Toxicity
In a series of experiments, sexually mature male rats received a single high dose (600 mg/kg) of DMH. Results showed a marked decline in both testis and thymus weights within seven days post-exposure. The study highlighted that repeated exposure (200 mg/kg/day for four weeks) led to severe testicular toxicity compared to structurally similar aliphatic ethers, indicating DMH's unique harmful effects on spermatogenesis .
Parameter | Control Group | DMH Treated Group | Change Observed |
---|---|---|---|
Testis Weight (g) | 1.5 | 0.45 | -70% |
Thymus Weight (g) | 0.25 | 0.13 | -48% |
Serum LDH (U/L) | 250 | 150 | -40% |
Uric Acid (mg/dL) | 5 | 2 | -60% |
Fuel Additive Research
Potential as Diesel Fuel Additive
DMH has been proposed as a candidate for use as a diesel fuel additive due to its properties that may enhance fuel performance while potentially reducing emissions. Its chemical structure allows it to function effectively within fuel mixtures, promoting better combustion characteristics .
Study on Fuel Additive Efficacy
Research conducted on the potential of DMH as a fuel additive indicated that it could improve the cetane number of diesel fuels, which is crucial for ignition quality. However, its toxicity profile raises concerns regarding its widespread use in commercial applications .
Chemical Synthesis Applications
Synthesis of Polyether Compounds
DMH serves as a precursor in the synthesis of polyether compounds, which have applications in various industries including pharmaceuticals and materials science. Its ability to undergo reactions such as etherification makes it valuable for producing more complex molecules .
作用机制
The mechanism of action of 1,6-dimethoxyhexane involves its interaction with nucleophiles and oxidizing agents. The ether linkages in the molecule are susceptible to nucleophilic attack, leading to substitution reactions. Additionally, the compound can undergo oxidation, where the ether groups are converted to carbonyl-containing functional groups .
相似化合物的比较
Similar Compounds
- 1,6-Hexanediol Dimethyl Ether
- Hexamethylene Glycol Dimethyl Ether
- Hexane, 1,1-dimethoxy-
Uniqueness
1,6-Dimethoxyhexane is unique due to its specific structure, which allows for distinct reactivity patterns compared to other dimethyl ethers. Its longer carbon chain and terminal ether groups make it particularly useful in applications requiring high boiling points and stability .
生物活性
1,6-Dimethoxyhexane (DMH) is an aliphatic ether that has garnered attention in toxicological studies due to its biological activity and potential health effects. This article explores the biological activity of DMH, focusing on its toxicity, mechanisms of action, and implications for human health and safety.
Chemical Structure and Properties
This compound is a linear ether with the molecular formula C8H18O2. Its structure features two methoxy groups attached to a hexane backbone. This configuration contributes to its solubility and reactivity, influencing its biological interactions.
Testicular Toxicity
One of the most significant findings regarding DMH is its testicular toxicity. Studies have shown that DMH exposure leads to substantial reductions in testis weight and alterations in spermatogenesis. In a study involving male Sprague-Dawley rats, high doses of DMH (200 mg/kg) resulted in a reduction of testis weight by approximately 70% compared to control groups . Histopathological examinations revealed degeneration of seminiferous tubules and reduced sperm density, indicating severe reproductive toxicity .
The biological activity of DMH is hypothesized to be mediated through its metabolic conversion to methoxyacetic acid (MAA), which is implicated in histone deacetylase (HDAC) inhibition. This inhibition can lead to altered gene expression related to cell cycle regulation and apoptosis . Increased histone acetylation due to HDAC inhibition may disrupt normal cellular processes, contributing to the observed toxic effects.
Summary of Findings from Case Studies
Short-term Oral Toxicity Studies
In short-term oral toxicity studies, DMH was administered over four weeks with varying doses. The results indicated:
- High Dose Effects : At 200 mg/kg, notable decreases in serum lactate dehydrogenase (LDH), uric acid levels, and blood platelet counts were observed .
- Biochemical Changes : Urinary ascorbic acid levels increased significantly across all treatment groups, suggesting altered metabolic pathways due to DMH exposure .
- Histological Changes : Mild histological changes were noted in the liver across all dose levels, indicating potential hepatotoxicity alongside reproductive effects .
Implications for Human Health
Given the observed toxicological effects in animal models, there are concerns regarding the potential risks associated with human exposure to DMH. The reproductive toxicity highlighted in studies suggests that DMH may pose significant risks for male fertility if similar effects are observed in humans.
属性
IUPAC Name |
1,6-dimethoxyhexane | |
---|---|---|
Source | PubChem | |
URL | https://pubchem.ncbi.nlm.nih.gov | |
Description | Data deposited in or computed by PubChem | |
InChI |
InChI=1S/C8H18O2/c1-9-7-5-3-4-6-8-10-2/h3-8H2,1-2H3 | |
Source | PubChem | |
URL | https://pubchem.ncbi.nlm.nih.gov | |
Description | Data deposited in or computed by PubChem | |
InChI Key |
GZHQUHIMIVEQFV-UHFFFAOYSA-N | |
Source | PubChem | |
URL | https://pubchem.ncbi.nlm.nih.gov | |
Description | Data deposited in or computed by PubChem | |
Canonical SMILES |
COCCCCCCOC | |
Source | PubChem | |
URL | https://pubchem.ncbi.nlm.nih.gov | |
Description | Data deposited in or computed by PubChem | |
Molecular Formula |
C8H18O2 | |
Source | PubChem | |
URL | https://pubchem.ncbi.nlm.nih.gov | |
Description | Data deposited in or computed by PubChem | |
DSSTOX Substance ID |
DTXSID30337391 | |
Record name | 1,6-Dimethoxyhexane | |
Source | EPA DSSTox | |
URL | https://comptox.epa.gov/dashboard/DTXSID30337391 | |
Description | DSSTox provides a high quality public chemistry resource for supporting improved predictive toxicology. | |
Molecular Weight |
146.23 g/mol | |
Source | PubChem | |
URL | https://pubchem.ncbi.nlm.nih.gov | |
Description | Data deposited in or computed by PubChem | |
CAS No. |
13179-98-1 | |
Record name | 1,6-Dimethoxyhexane | |
Source | CAS Common Chemistry | |
URL | https://commonchemistry.cas.org/detail?cas_rn=13179-98-1 | |
Description | CAS Common Chemistry is an open community resource for accessing chemical information. Nearly 500,000 chemical substances from CAS REGISTRY cover areas of community interest, including common and frequently regulated chemicals, and those relevant to high school and undergraduate chemistry classes. This chemical information, curated by our expert scientists, is provided in alignment with our mission as a division of the American Chemical Society. | |
Explanation | The data from CAS Common Chemistry is provided under a CC-BY-NC 4.0 license, unless otherwise stated. | |
Record name | 1,6-Dimethoxyhexane | |
Source | ChemIDplus | |
URL | https://pubchem.ncbi.nlm.nih.gov/substance/?source=chemidplus&sourceid=0013179981 | |
Description | ChemIDplus is a free, web search system that provides access to the structure and nomenclature authority files used for the identification of chemical substances cited in National Library of Medicine (NLM) databases, including the TOXNET system. | |
Record name | 1,6-Dimethoxyhexane | |
Source | EPA DSSTox | |
URL | https://comptox.epa.gov/dashboard/DTXSID30337391 | |
Description | DSSTox provides a high quality public chemistry resource for supporting improved predictive toxicology. | |
Record name | Hexane, 1,6-dimethoxy | |
Source | European Chemicals Agency (ECHA) | |
URL | https://echa.europa.eu/information-on-chemicals | |
Description | The European Chemicals Agency (ECHA) is an agency of the European Union which is the driving force among regulatory authorities in implementing the EU's groundbreaking chemicals legislation for the benefit of human health and the environment as well as for innovation and competitiveness. | |
Explanation | Use of the information, documents and data from the ECHA website is subject to the terms and conditions of this Legal Notice, and subject to other binding limitations provided for under applicable law, the information, documents and data made available on the ECHA website may be reproduced, distributed and/or used, totally or in part, for non-commercial purposes provided that ECHA is acknowledged as the source: "Source: European Chemicals Agency, http://echa.europa.eu/". Such acknowledgement must be included in each copy of the material. ECHA permits and encourages organisations and individuals to create links to the ECHA website under the following cumulative conditions: Links can only be made to webpages that provide a link to the Legal Notice page. | |
Retrosynthesis Analysis
AI-Powered Synthesis Planning: Our tool employs the Template_relevance Pistachio, Template_relevance Bkms_metabolic, Template_relevance Pistachio_ringbreaker, Template_relevance Reaxys, Template_relevance Reaxys_biocatalysis model, leveraging a vast database of chemical reactions to predict feasible synthetic routes.
One-Step Synthesis Focus: Specifically designed for one-step synthesis, it provides concise and direct routes for your target compounds, streamlining the synthesis process.
Accurate Predictions: Utilizing the extensive PISTACHIO, BKMS_METABOLIC, PISTACHIO_RINGBREAKER, REAXYS, REAXYS_BIOCATALYSIS database, our tool offers high-accuracy predictions, reflecting the latest in chemical research and data.
Strategy Settings
Precursor scoring | Relevance Heuristic |
---|---|
Min. plausibility | 0.01 |
Model | Template_relevance |
Template Set | Pistachio/Bkms_metabolic/Pistachio_ringbreaker/Reaxys/Reaxys_biocatalysis |
Top-N result to add to graph | 6 |
Feasible Synthetic Routes
Q1: What are the primary toxicological concerns associated with 1,6-dimethoxyhexane?
A: Research indicates that this compound exhibits significant testicular toxicity in animal models. [, ] This toxicity manifests as a reduction in testis and thymus weight, degeneration of seminiferous tubules, and decreased sperm density. [, ] Evidence suggests this toxicity stems from its metabolism into methoxyacetic acid (MAA), a known testicular toxicant. [, ]
Q2: How does this compound interact with biological systems at the cellular level?
A: While this compound itself might not directly interact with cellular components, its metabolite, methoxyacetic acid (MAA), is believed to exert toxicity by interfering with histone deacetylase activity. [] This interference disrupts critical cellular processes, particularly in rapidly dividing cells like those in the testes, leading to the observed testicular toxicity.
Q3: Beyond toxicity, what other properties of this compound have been investigated?
A: Researchers have explored the conformational behavior of this compound in liquid crystal environments using techniques like deuterium NMR spectroscopy. [, ] These studies reveal that the molecule exhibits conformational flexibility and can adopt different spatial arrangements depending on the surrounding environment.
体外研究产品的免责声明和信息
请注意,BenchChem 上展示的所有文章和产品信息仅供信息参考。 BenchChem 上可购买的产品专为体外研究设计,这些研究在生物体外进行。体外研究,源自拉丁语 "in glass",涉及在受控实验室环境中使用细胞或组织进行的实验。重要的是要注意,这些产品没有被归类为药物或药品,他们没有得到 FDA 的批准,用于预防、治疗或治愈任何医疗状况、疾病或疾病。我们必须强调,将这些产品以任何形式引入人类或动物的身体都是法律严格禁止的。遵守这些指南对确保研究和实验的法律和道德标准的符合性至关重要。