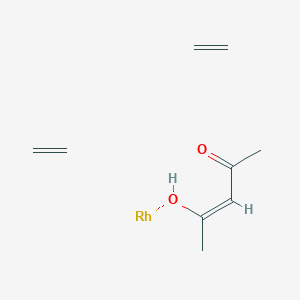
乙酰丙酮双(乙烯)铑(I)
- 点击 快速询问 获取最新报价。
- 提供有竞争力价格的高质量产品,您可以更专注于研究。
描述
Acetylacetonatobis(ethylene)rhodium(I) is a coordination compound commonly used as a precursor to synthesize mononuclear rhodium complexes. It is also employed as a catalyst in asymmetric arylation reactions . The compound has the empirical formula C9H15O2Rh and a molecular weight of 258.12 g/mol .
科学研究应用
Acetylacetonatobis(ethylene)rhodium(I) has a wide range of applications in scientific research:
Biology: The compound is studied for its potential biological activity and interactions with biomolecules.
Medicine: Research is ongoing to explore its potential use in medicinal chemistry, particularly in the development of new drugs.
准备方法
Synthetic Routes and Reaction Conditions
Acetylacetonatobis(ethylene)rhodium(I) is typically synthesized by reacting rhodium trichloride with acetylacetone and ethylene under controlled conditions. The reaction involves the formation of a rhodium-acetylacetonate complex, which is then treated with ethylene to yield the final product .
Industrial Production Methods
While specific industrial production methods are not widely documented, the synthesis generally follows the laboratory preparation route, scaled up to meet industrial demands. The reaction conditions are optimized to ensure high yield and purity of the compound .
化学反应分析
Types of Reactions
Acetylacetonatobis(ethylene)rhodium(I) undergoes various types of reactions, including:
Oxidation: The compound can be oxidized to form higher oxidation state rhodium complexes.
Reduction: It can be reduced to form lower oxidation state rhodium species.
Substitution: The ethylene ligands can be substituted with other ligands, such as phosphines or amines.
Common Reagents and Conditions
Oxidation: Common oxidizing agents include oxygen or peroxides.
Reduction: Reducing agents such as hydrogen or hydrides are used.
Substitution: Ligand substitution reactions often involve the use of phosphines or amines under mild conditions.
Major Products Formed
Oxidation: Higher oxidation state rhodium complexes.
Reduction: Lower oxidation state rhodium species.
Substitution: Rhodium complexes with substituted ligands.
作用机制
The mechanism by which Acetylacetonatobis(ethylene)rhodium(I) exerts its effects involves the coordination of the rhodium center with various ligands. The ethylene and acetylacetonate ligands stabilize the rhodium center, allowing it to participate in catalytic cycles. The compound can facilitate the transfer of electrons and protons, enabling various chemical transformations .
相似化合物的比较
Similar Compounds
- Bis(ethylene)rhodium(I) acetylacetonate
- Diethylene (acetylacetonato)rhodium
- Bis(η2-ethene)(2,4-pentanedionato-κO,κO′)rhodium
Uniqueness
Acetylacetonatobis(ethylene)rhodium(I) is unique due to its specific combination of ethylene and acetylacetonate ligands, which provide a balance of stability and reactivity. This makes it particularly effective as a catalyst in asymmetric arylation reactions, distinguishing it from other rhodium complexes .
属性
CAS 编号 |
12082-47-2 |
---|---|
分子式 |
C9H15O2Rh- |
分子量 |
258.12 g/mol |
IUPAC 名称 |
ethene;pentane-2,4-dione;rhodium |
InChI |
InChI=1S/C5H7O2.2C2H4.Rh/c1-4(6)3-5(2)7;2*1-2;/h3H,1-2H3;2*1-2H2;/q-1;;; |
InChI 键 |
FUBJEWKSRADMDM-UHFFFAOYSA-N |
SMILES |
CC(=CC(=O)C)O.C=C.C=C.[Rh] |
手性 SMILES |
C/C(=C/C(=O)C)/[O-].C=C.C=C.[Rh] |
规范 SMILES |
CC(=O)[CH-]C(=O)C.C=C.C=C.[Rh] |
Pictograms |
Irritant |
产品来源 |
United States |
Retrosynthesis Analysis
AI-Powered Synthesis Planning: Our tool employs the Template_relevance Pistachio, Template_relevance Bkms_metabolic, Template_relevance Pistachio_ringbreaker, Template_relevance Reaxys, Template_relevance Reaxys_biocatalysis model, leveraging a vast database of chemical reactions to predict feasible synthetic routes.
One-Step Synthesis Focus: Specifically designed for one-step synthesis, it provides concise and direct routes for your target compounds, streamlining the synthesis process.
Accurate Predictions: Utilizing the extensive PISTACHIO, BKMS_METABOLIC, PISTACHIO_RINGBREAKER, REAXYS, REAXYS_BIOCATALYSIS database, our tool offers high-accuracy predictions, reflecting the latest in chemical research and data.
Strategy Settings
Precursor scoring | Relevance Heuristic |
---|---|
Min. plausibility | 0.01 |
Model | Template_relevance |
Template Set | Pistachio/Bkms_metabolic/Pistachio_ringbreaker/Reaxys/Reaxys_biocatalysis |
Top-N result to add to graph | 6 |
Feasible Synthetic Routes
Q1: What are the catalytic applications of Acetylacetonatobis(ethylene)rhodium(I) and how does its structure contribute to its activity?
A1: Acetylacetonatobis(ethylene)rhodium(I), often abbreviated as [(acac)Rh(C2H4)2], is a valuable catalyst in various organic reactions. Its structure plays a crucial role in its catalytic properties []:
Q2: Are there any safety concerns associated with handling Acetylacetonatobis(ethylene)rhodium(I) in a laboratory setting?
A2: Yes, safety precautions are crucial when handling Acetylacetonatobis(ethylene)rhodium(I) []:
体外研究产品的免责声明和信息
请注意,BenchChem 上展示的所有文章和产品信息仅供信息参考。 BenchChem 上可购买的产品专为体外研究设计,这些研究在生物体外进行。体外研究,源自拉丁语 "in glass",涉及在受控实验室环境中使用细胞或组织进行的实验。重要的是要注意,这些产品没有被归类为药物或药品,他们没有得到 FDA 的批准,用于预防、治疗或治愈任何医疗状况、疾病或疾病。我们必须强调,将这些产品以任何形式引入人类或动物的身体都是法律严格禁止的。遵守这些指南对确保研究和实验的法律和道德标准的符合性至关重要。