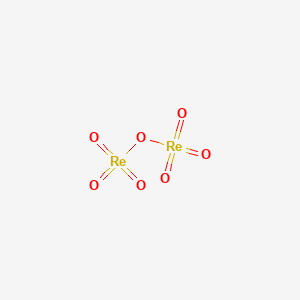
七氧化铼
描述
Rhenium(VII) oxide, also known as dirhenium heptoxide, is an inorganic compound with the chemical formula Re₂O₇. This yellow crystalline solid is the anhydride of perrhenic acid and serves as a precursor to various rhenium compounds. It is a significant material in the field of inorganic chemistry due to its unique properties and applications .
科学研究应用
Rhenium(VII) oxide has a wide range of applications in scientific research:
Biology and Medicine: Investigated for potential use in radiotherapy due to its radioactive isotopes.
安全和危害
Rhenium(VII) oxide is considered hazardous. It can cause skin irritation, serious eye irritation, and may cause respiratory irritation . It is advised to avoid dust formation, breathing vapors, mist, or gas, and to ensure adequate ventilation . It is also recommended to use personal protective equipment .
未来方向
作用机制
Target of Action
Rhenium(VII) oxide, also known as dirhenium heptoxide, primarily targets organic compounds, particularly those containing carbon-carbon double bonds . It acts as a catalyst in various organic reactions .
Mode of Action
Rhenium(VII) oxide interacts with its targets through oxidation and metathesis reactions . It can catalyze the oxidation of olefins and facilitate the rearrangement of allylic alcohols, oximes, and amides . The compound’s mode of action involves the formation of unstable rhenium(VII) alkoxides . Rhenium(VII) oxidizes the alkoxy group while being reduced to a lower oxidation number, predominantly to +6 .
Biochemical Pathways
It is known to be involved in oxidative cyclizations , alkene metathesis , and the formation of bisperoxyacetals . These reactions can lead to various downstream effects, including the synthesis of complex organic compounds.
Pharmacokinetics
It is a yellow crystalline powder that is soluble in water, forming perrhenic acid . This solubility can impact its bioavailability in aqueous environments.
Result of Action
The primary result of Rhenium(VII) oxide’s action is the transformation of organic compounds. It can facilitate the dehydration and rearrangement of certain compounds , and it can catalyze the formation of new compounds through oxidative cyclizations and alkene metathesis .
Action Environment
The action of Rhenium(VII) oxide is influenced by environmental factors such as temperature and the presence of other compounds. It is formed when metallic rhenium or its oxides or sulfides are oxidized at 500–700 °C (900–1,300 °F) in air . The compound’s reactivity can also be affected by the presence of other substances, such as tetramethyltin, with which it reacts to form methylrhenium trioxide .
生化分析
Biochemical Properties
Rhenium(VII) oxide acts as a precursor to organorhenium oxides, which are known to catalyze olefin oxidation, metathesis, and other transformations . These reactions involve the interaction of Rhenium(VII) oxide with various enzymes and proteins, leading to significant biochemical changes.
Molecular Mechanism
The molecular mechanism of Rhenium(VII) oxide involves its transformation into organorhenium oxides, which then interact with biomolecules to catalyze various reactions . These interactions can lead to changes in gene expression and enzyme activity.
准备方法
Synthetic Routes and Reaction Conditions: Rhenium(VII) oxide is typically synthesized by oxidizing metallic rhenium or its oxides or sulfides at temperatures ranging from 500 to 700°C in the presence of air . Another method involves the reaction of rhenium metal with hydrogen peroxide to form perrhenic acid, which is then dehydrated to yield rhenium(VII) oxide .
Industrial Production Methods: In industrial settings, rhenium(VII) oxide is produced by roasting rhenium-containing ores, such as molybdenite, in an oxidizing atmosphere. The volatile rhenium(VII) oxide is then collected and purified .
Types of Reactions:
Substitution: Rhenium(VII) oxide reacts with organometallic compounds to form organorhenium oxides, which are useful in catalysis.
Common Reagents and Conditions:
Tetramethyltin: Reacts with rhenium(VII) oxide to form methylrhenium trioxide, a catalyst for oxidation reactions.
Hexamethyldisiloxane: Reacts to form siloxide derivatives.
Major Products:
Rhenium Dioxide (ReO₂): Formed by heating rhenium(VII) oxide.
Methylrhenium Trioxide: Formed by reaction with tetramethyltin.
相似化合物的比较
- Manganese(VII) oxide (Mn₂O₇)
- Technetium(VII) oxide (Tc₂O₇)
- Perrhenic acid (HReO₄)
Comparison: Rhenium(VII) oxide is unique due to its high stability and ability to form a wide range of organorhenium compounds. Unlike manganese(VII) oxide and technetium(VII) oxide, rhenium(VII) oxide is more stable and less reactive, making it more suitable for industrial applications .
属性
IUPAC Name |
trioxo(trioxorheniooxy)rhenium | |
---|---|---|
Source | PubChem | |
URL | https://pubchem.ncbi.nlm.nih.gov | |
Description | Data deposited in or computed by PubChem | |
InChI |
InChI=1S/7O.2Re | |
Source | PubChem | |
URL | https://pubchem.ncbi.nlm.nih.gov | |
Description | Data deposited in or computed by PubChem | |
InChI Key |
NBGOSNNAAHDRLK-UHFFFAOYSA-N | |
Source | PubChem | |
URL | https://pubchem.ncbi.nlm.nih.gov | |
Description | Data deposited in or computed by PubChem | |
Canonical SMILES |
O=[Re](=O)(=O)O[Re](=O)(=O)=O | |
Source | PubChem | |
URL | https://pubchem.ncbi.nlm.nih.gov | |
Description | Data deposited in or computed by PubChem | |
Molecular Formula |
Re2O7, O7Re2 | |
Record name | Rhenium(VII) oxide | |
Source | Wikipedia | |
URL | https://en.wikipedia.org/wiki/Rhenium(VII)_oxide | |
Description | Chemical information link to Wikipedia. | |
Source | PubChem | |
URL | https://pubchem.ncbi.nlm.nih.gov | |
Description | Data deposited in or computed by PubChem | |
DSSTOX Substance ID |
DTXSID00894901 | |
Record name | Dirhenium heptaoxide | |
Source | EPA DSSTox | |
URL | https://comptox.epa.gov/dashboard/DTXSID00894901 | |
Description | DSSTox provides a high quality public chemistry resource for supporting improved predictive toxicology. | |
Molecular Weight |
484.41 g/mol | |
Source | PubChem | |
URL | https://pubchem.ncbi.nlm.nih.gov | |
Description | Data deposited in or computed by PubChem | |
Physical Description |
Canary yellow solid; Highly deliquescent; [Merck Index] Yellow or greenish-yellow powder; [MSDSonline] | |
Record name | Rhenium heptoxide | |
Source | Haz-Map, Information on Hazardous Chemicals and Occupational Diseases | |
URL | https://haz-map.com/Agents/8676 | |
Description | Haz-Map® is an occupational health database designed for health and safety professionals and for consumers seeking information about the adverse effects of workplace exposures to chemical and biological agents. | |
Explanation | Copyright (c) 2022 Haz-Map(R). All rights reserved. Unless otherwise indicated, all materials from Haz-Map are copyrighted by Haz-Map(R). No part of these materials, either text or image may be used for any purpose other than for personal use. Therefore, reproduction, modification, storage in a retrieval system or retransmission, in any form or by any means, electronic, mechanical or otherwise, for reasons other than personal use, is strictly prohibited without prior written permission. | |
CAS No. |
1314-68-7 | |
Record name | Rhenium heptoxide | |
Source | ChemIDplus | |
URL | https://pubchem.ncbi.nlm.nih.gov/substance/?source=chemidplus&sourceid=0001314687 | |
Description | ChemIDplus is a free, web search system that provides access to the structure and nomenclature authority files used for the identification of chemical substances cited in National Library of Medicine (NLM) databases, including the TOXNET system. | |
Record name | Rhenium oxide (Re2O7) | |
Source | EPA Chemicals under the TSCA | |
URL | https://www.epa.gov/chemicals-under-tsca | |
Description | EPA Chemicals under the Toxic Substances Control Act (TSCA) collection contains information on chemicals and their regulations under TSCA, including non-confidential content from the TSCA Chemical Substance Inventory and Chemical Data Reporting. | |
Record name | Dirhenium heptaoxide | |
Source | EPA DSSTox | |
URL | https://comptox.epa.gov/dashboard/DTXSID00894901 | |
Description | DSSTox provides a high quality public chemistry resource for supporting improved predictive toxicology. | |
Record name | Dirhenium heptaoxide | |
Source | European Chemicals Agency (ECHA) | |
URL | https://echa.europa.eu/substance-information/-/substanceinfo/100.013.857 | |
Description | The European Chemicals Agency (ECHA) is an agency of the European Union which is the driving force among regulatory authorities in implementing the EU's groundbreaking chemicals legislation for the benefit of human health and the environment as well as for innovation and competitiveness. | |
Explanation | Use of the information, documents and data from the ECHA website is subject to the terms and conditions of this Legal Notice, and subject to other binding limitations provided for under applicable law, the information, documents and data made available on the ECHA website may be reproduced, distributed and/or used, totally or in part, for non-commercial purposes provided that ECHA is acknowledged as the source: "Source: European Chemicals Agency, http://echa.europa.eu/". Such acknowledgement must be included in each copy of the material. ECHA permits and encourages organisations and individuals to create links to the ECHA website under the following cumulative conditions: Links can only be made to webpages that provide a link to the Legal Notice page. | |
Record name | RHENIUM HEPTOXIDE | |
Source | FDA Global Substance Registration System (GSRS) | |
URL | https://gsrs.ncats.nih.gov/ginas/app/beta/substances/0DJ421156F | |
Description | The FDA Global Substance Registration System (GSRS) enables the efficient and accurate exchange of information on what substances are in regulated products. Instead of relying on names, which vary across regulatory domains, countries, and regions, the GSRS knowledge base makes it possible for substances to be defined by standardized, scientific descriptions. | |
Explanation | Unless otherwise noted, the contents of the FDA website (www.fda.gov), both text and graphics, are not copyrighted. They are in the public domain and may be republished, reprinted and otherwise used freely by anyone without the need to obtain permission from FDA. Credit to the U.S. Food and Drug Administration as the source is appreciated but not required. | |
ANone: The interaction of Rhenium(VII) oxide with its target depends heavily on the application.
- In olefin metathesis: Re₂O₇, often supported on alumina, reacts with olefins to form metallacyclobutane intermediates. [, ] These intermediates then decompose to regenerate the catalyst and produce new olefins, enabling reactions like alkene self-metathesis and cross-metathesis. []
- In dehydration reactions: Re₂O₇ acts as a Lewis acid, coordinating to the oxygen atom of the alcohol. [, , ] This interaction weakens the C-O bond, facilitating the elimination of water and the formation of an olefin. [, , ]
- In oxidative cyclizations: Re₂O₇ activates bis-homoallylic alcohols, promoting their cyclization into poly-tetrahydrofuran (THF) structures. [, , ] This interaction is highly stereoselective, leading to predictable product configurations. [, , ]
A: Rhenium(VII) oxide (Re₂O₇) is a yellow solid with a melting point of 297–300 °C and a boiling point of 360 °C. []
- Spectroscopic data:
- IR Spectroscopy: Shows distinct bands for different surface rhenium species, such as [ReO₄]ads− and [Re₂O₇]ads, when supported on alumina. []
- Raman Spectroscopy: Confirms structural differences between dehydrated and hydrated forms of Re₂O₇ on alumina. []
- Nuclear Quadrupole Resonance (NQR): Reveals a strong asymmetry in the electronic charge distribution around the central rhenium atom due to bridging oxygen atoms. []
A: Rhenium(VII) oxide is highly soluble in aprotic donor solvents like acetonitrile, tetrahydrofuran, and pyridine, and modestly soluble in other organic solvents. [] It is hygroscopic, readily forming perrhenic acid (HReO₄) upon contact with water. []
- Stability: Re₂O₇ is thermally stable but decomposes in the presence of reducing agents. [] It can cleave tetrahydrofuran in the presence of trifluoroacetic anhydride and a carboxylic acid. []
- Applications under various conditions:
- Dehydration reactions: Effective for a wide range of alcohols (allylic, aliphatic, homoallylic, tertiary, and secondary) at relatively mild conditions (100–150 °C, ambient atmosphere) in technical toluene. []
- Olefin metathesis: Typically used at elevated temperatures on an alumina support. [, ] The activity depends on the loading of Re₂O₇, with higher loadings leading to greater activity. [, ]
- Oxidative cyclizations: Often carried out in chlorinated solvents like dichloromethane. [] Requires careful control of reaction conditions to achieve desired selectivity. []
ANone: Rhenium(VII) oxide is a versatile catalyst used in various organic reactions.
- Alkene Metathesis: Re₂O₇ supported on alumina is a well-known heterogeneous catalyst for olefin metathesis. [, , ]
- Mechanism: Involves a pseudo-Wittig initiation step where a metallaoxacyclobutane intermediate is formed. [] This intermediate then undergoes cycloreversion to generate new olefins. []
- Selectivity: Can catalyze both self-metathesis and cross-metathesis of olefins. [] The selectivity depends on the reaction conditions and the nature of the olefins. []
- Dehydration of Alcohols: Re₂O₇ is an efficient and selective catalyst for dehydrating various alcohols to their corresponding olefins. [, ]
- Oxidative Polycyclizations: Re₂O₇ promotes the oxidative cyclization of polyenes containing bis-homoallylic alcohols to form poly-THF products. [, , ]
ANone: While the provided abstracts do not explicitly discuss detailed computational studies on Re₂O₇, such studies are certainly relevant and likely conducted in the broader research landscape.
- DFT Calculations: Density functional theory (DFT) calculations are valuable for studying the electronic structure and bonding in Re₂O₇ and its complexes. [, ] They can provide insights into the reaction mechanisms and origins of selectivity in Re₂O₇-catalyzed reactions.
ANone: While the abstracts primarily focus on Re₂O₇ itself, understanding the SAR is crucial for developing improved catalysts.
- Ligand Modification: Introducing different ligands around the rhenium center can significantly alter its Lewis acidity, steric environment, and consequently its catalytic activity and selectivity. [, , ] For instance, bulky ligands can influence the diastereoselectivity of oxidative cyclizations. []
- Support Effects: In heterogeneous catalysis, the support material plays a vital role. [, , ] The interaction between Re₂O₇ and the support (e.g., alumina) can influence the dispersion, oxidation state, and catalytic properties of the active rhenium species. [, , ]
ANone:
- Molybdenum-based catalysts: These offer a potentially more cost-effective alternative to Re₂O₇, showing intermediate activity and selectivity in dehydration reactions. [] They have also demonstrated good activity in lactic acid deoxygenation to propionic acid. []
- Traditional Brønsted acids: Sulfuric acid, for example, is a common dehydration catalyst but often suffers from lower selectivity and environmental concerns compared to Re₂O₇. []
- Solid acid catalysts: Acid resins, zeolites, and mesoporous materials offer heterogeneity but are often outperformed by Re₂O₇ in terms of activity and selectivity for dehydration reactions. []
ANone: While the provided abstracts do not offer a historical overview, key milestones likely include:
ANone: Rhenium(VII) oxide's unique properties lend themselves to cross-disciplinary research:
- Catalysis and Materials Science: The use of Re₂O₇ as a catalyst in various organic reactions intersects with materials science, particularly in designing and developing heterogeneous catalysts with improved activity, selectivity, and stability. [, , ]
- Organic Synthesis and Natural Product Chemistry: The application of Re₂O₇ in oxidative polycyclizations has significant implications for synthesizing complex natural products, such as Annonaceous acetogenins. [, , ]
- Sustainable Chemistry and Biomass Conversion: Re₂O₇'s effectiveness in dehydrating bio-based alcohols like terpenes and lactic acid highlights its potential in biomass conversion and developing sustainable chemical processes. [, ]
体外研究产品的免责声明和信息
请注意,BenchChem 上展示的所有文章和产品信息仅供信息参考。 BenchChem 上可购买的产品专为体外研究设计,这些研究在生物体外进行。体外研究,源自拉丁语 "in glass",涉及在受控实验室环境中使用细胞或组织进行的实验。重要的是要注意,这些产品没有被归类为药物或药品,他们没有得到 FDA 的批准,用于预防、治疗或治愈任何医疗状况、疾病或疾病。我们必须强调,将这些产品以任何形式引入人类或动物的身体都是法律严格禁止的。遵守这些指南对确保研究和实验的法律和道德标准的符合性至关重要。