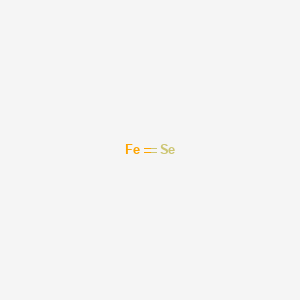
硒化铁
描述
Iron selenide is an inorganic compound composed of iron and selenium. It is known for its unique properties, including its role as an unconventional iron-based low-temperature superconductor. Iron selenide exhibits zero electrical resistance when a current is passed through it, making it a subject of interest in the field of superconductivity . Additionally, iron selenide has a narrow band-gap and high absorption coefficient, which makes it suitable for applications in solar cells .
科学研究应用
Iron selenide has a wide range of scientific research applications:
Superconductivity: Iron selenide is studied for its superconducting properties, particularly its ability to exhibit zero electrical resistance at low temperatures.
Solar Cells: Due to its narrow band-gap and high absorption coefficient, iron selenide is used as a material in solar cells.
Electrocatalysis: Iron selenide is used as an electrocatalyst in water splitting reactions, including oxygen evolution and hydrogen evolution reactions.
Energy Storage: Iron selenide particles are used in supercapacitors due to their high capacitance and energy density.
Bioimaging: Iron selenide nanomaterials are explored for their potential use in bioimaging applications.
作用机制
Target of Action
Iron selenide, also known as Iron(II) selenide, is a compound that has been extensively studied for its unique properties in various fields . The primary targets of iron selenide are the electron transport systems in various applications, such as superconductors, semiconductors, and energy storage systems .
Biochemical Pathways
Iron selenide interacts with biochemical pathways primarily through its selenide component . Selenium is an essential trace element for human beings and animals, playing a critical role in human and animal health via various selenoproteins . Algae can absorb inorganic selenium from water and reduce it to selenoamino acids via the sulfur reduction pathway, which is similar to the selenium metabolism pathway in plants .
Pharmacokinetics
It’s worth noting that the primary site of action of iron is the erythrocyte, and no drug-receptor interaction takes place . The process of erythropoiesis, i.e., formation of new erythrocytes, takes 3-4 weeks .
Result of Action
The result of iron selenide’s action is primarily observed in its electrical properties . For instance, in potassium-ion batteries (KIBs), iron selenide composite in ether-based electrolyte presents a homogeneous electrochemical reaction, resulting in a stable potassium-ion storage . It presents a reversible specific capacity of 356 mAh g−1 at 200 mA g−1 after 75 cycles .
Action Environment
The action of iron selenide is influenced by environmental factors. For example, in the case of KIBs, iron selenide composite matching ether-based electrolyte presents a few minor morphology changes, indicating an occurrence of homogeneous electrochemical reaction in ether-based electrolyte, which results in a stable performance for potassium-ion (K-ion) storage . This suggests that the choice of electrolyte can significantly influence the action, efficacy, and stability of iron selenide.
生化分析
Cellular Effects
Some studies suggest that Iron selenide nanoparticles have shown potential in electrocatalysis water splitting
Molecular Mechanism
Some studies suggest that the phase determining factor in the synthesis of Iron selenide nanocrystals is the coordinating reactivity difference between olefins, tributylphosphine, and trioctylphosphine associated with their corresponding Se bond cleavage
Temporal Effects in Laboratory Settings
Some studies suggest that Iron selenide exhibits high capacitance and energy density, as well as extremely high cycling stability
Dosage Effects in Animal Models
While selenium, a component of Iron selenide, is known to have beneficial effects on animal health
Metabolic Pathways
Some studies suggest that selenium, a component of Iron selenide, is involved in various metabolic processes
Transport and Distribution
Some studies suggest that Iron selenide exhibits high electrochemical properties , which may influence its transport and distribution
Subcellular Localization
While some studies suggest that selenium, a component of Iron selenide, can be localized in various subcellular compartments
准备方法
Iron selenide can be synthesized through various methods, including:
Solid State Reactions: This involves heating iron and selenium powders in a sealed tube at high temperatures.
Chemical Vapor Deposition: This method involves the reaction of iron and selenium vapors on a substrate to form thin films.
Solution Chemistry Routes: Iron selenide nanocrystals can be synthesized by the thermal decomposition of ferrous chloride and selenium powder in solvents like oleylamine.
Chemical Vapor Transport: This method involves transporting the reactants in the vapor phase and depositing them onto a substrate.
Spray Pyrolysis: This technique involves spraying a solution of iron and selenium precursors onto a heated substrate to form iron selenide films.
化学反应分析
Iron selenide undergoes various chemical reactions, including:
Oxidation: Iron selenide can be oxidized to form iron oxides and selenium dioxide.
Reduction: It can be reduced to elemental iron and selenium under certain conditions.
Substitution: Iron selenide can undergo substitution reactions where selenium atoms are replaced by other chalcogens like sulfur.
Common reagents used in these reactions include hydrogen peroxide for oxidation and hydrazine for reduction. The major products formed from these reactions depend on the specific conditions and reagents used.
相似化合物的比较
Iron selenide can be compared with other similar compounds, such as:
Iron Sulfide: Like iron selenide, iron sulfide is also a chalcogenide with similar properties but differs in its band-gap and reactivity.
Iron Telluride: This compound is another iron chalcogenide with different superconducting properties and applications.
Nickel Selenide: Nickel selenide shares some electrocatalytic properties with iron selenide but has different electronic and structural characteristics.
Iron selenide is unique due to its combination of superconducting properties, narrow band-gap, and high absorption coefficient, making it suitable for a wide range of applications.
属性
IUPAC Name |
selanylideneiron | |
---|---|---|
Source | PubChem | |
URL | https://pubchem.ncbi.nlm.nih.gov | |
Description | Data deposited in or computed by PubChem | |
InChI |
InChI=1S/Fe.Se | |
Source | PubChem | |
URL | https://pubchem.ncbi.nlm.nih.gov | |
Description | Data deposited in or computed by PubChem | |
InChI Key |
WALCGGIJOOWJIN-UHFFFAOYSA-N | |
Source | PubChem | |
URL | https://pubchem.ncbi.nlm.nih.gov | |
Description | Data deposited in or computed by PubChem | |
Canonical SMILES |
[Fe]=[Se] | |
Source | PubChem | |
URL | https://pubchem.ncbi.nlm.nih.gov | |
Description | Data deposited in or computed by PubChem | |
Molecular Formula |
FeSe | |
Record name | iron(II) selenide | |
Source | Wikipedia | |
URL | https://en.wikipedia.org/wiki/Iron(II)_selenide | |
Description | Chemical information link to Wikipedia. | |
Source | PubChem | |
URL | https://pubchem.ncbi.nlm.nih.gov | |
Description | Data deposited in or computed by PubChem | |
DSSTOX Substance ID |
DTXSID1061653 | |
Record name | Iron(II) selenide | |
Source | EPA DSSTox | |
URL | https://comptox.epa.gov/dashboard/DTXSID1061653 | |
Description | DSSTox provides a high quality public chemistry resource for supporting improved predictive toxicology. | |
Molecular Weight |
134.82 g/mol | |
Source | PubChem | |
URL | https://pubchem.ncbi.nlm.nih.gov | |
Description | Data deposited in or computed by PubChem | |
CAS No. |
1310-32-3, 50926-12-0 | |
Record name | Iron selenide (FeSe) | |
Source | CAS Common Chemistry | |
URL | https://commonchemistry.cas.org/detail?cas_rn=1310-32-3 | |
Description | CAS Common Chemistry is an open community resource for accessing chemical information. Nearly 500,000 chemical substances from CAS REGISTRY cover areas of community interest, including common and frequently regulated chemicals, and those relevant to high school and undergraduate chemistry classes. This chemical information, curated by our expert scientists, is provided in alignment with our mission as a division of the American Chemical Society. | |
Explanation | The data from CAS Common Chemistry is provided under a CC-BY-NC 4.0 license, unless otherwise stated. | |
Record name | Iron selenide | |
Source | CAS Common Chemistry | |
URL | https://commonchemistry.cas.org/detail?cas_rn=50926-12-0 | |
Description | CAS Common Chemistry is an open community resource for accessing chemical information. Nearly 500,000 chemical substances from CAS REGISTRY cover areas of community interest, including common and frequently regulated chemicals, and those relevant to high school and undergraduate chemistry classes. This chemical information, curated by our expert scientists, is provided in alignment with our mission as a division of the American Chemical Society. | |
Explanation | The data from CAS Common Chemistry is provided under a CC-BY-NC 4.0 license, unless otherwise stated. | |
Record name | Ferrous selenide | |
Source | ChemIDplus | |
URL | https://pubchem.ncbi.nlm.nih.gov/substance/?source=chemidplus&sourceid=0001310323 | |
Description | ChemIDplus is a free, web search system that provides access to the structure and nomenclature authority files used for the identification of chemical substances cited in National Library of Medicine (NLM) databases, including the TOXNET system. | |
Record name | 1310-32-3 | |
Source | DTP/NCI | |
URL | https://dtp.cancer.gov/dtpstandard/servlet/dwindex?searchtype=NSC&outputformat=html&searchlist=378337 | |
Description | The NCI Development Therapeutics Program (DTP) provides services and resources to the academic and private-sector research communities worldwide to facilitate the discovery and development of new cancer therapeutic agents. | |
Explanation | Unless otherwise indicated, all text within NCI products is free of copyright and may be reused without our permission. Credit the National Cancer Institute as the source. | |
Record name | Iron selenide (FeSe) | |
Source | EPA Chemicals under the TSCA | |
URL | https://www.epa.gov/chemicals-under-tsca | |
Description | EPA Chemicals under the Toxic Substances Control Act (TSCA) collection contains information on chemicals and their regulations under TSCA, including non-confidential content from the TSCA Chemical Substance Inventory and Chemical Data Reporting. | |
Record name | Iron(II) selenide | |
Source | EPA DSSTox | |
URL | https://comptox.epa.gov/dashboard/DTXSID1061653 | |
Description | DSSTox provides a high quality public chemistry resource for supporting improved predictive toxicology. | |
Record name | Iron selenide | |
Source | European Chemicals Agency (ECHA) | |
URL | https://echa.europa.eu/substance-information/-/substanceinfo/100.013.798 | |
Description | The European Chemicals Agency (ECHA) is an agency of the European Union which is the driving force among regulatory authorities in implementing the EU's groundbreaking chemicals legislation for the benefit of human health and the environment as well as for innovation and competitiveness. | |
Explanation | Use of the information, documents and data from the ECHA website is subject to the terms and conditions of this Legal Notice, and subject to other binding limitations provided for under applicable law, the information, documents and data made available on the ECHA website may be reproduced, distributed and/or used, totally or in part, for non-commercial purposes provided that ECHA is acknowledged as the source: "Source: European Chemicals Agency, http://echa.europa.eu/". Such acknowledgement must be included in each copy of the material. ECHA permits and encourages organisations and individuals to create links to the ECHA website under the following cumulative conditions: Links can only be made to webpages that provide a link to the Legal Notice page. | |
体外研究产品的免责声明和信息
请注意,BenchChem 上展示的所有文章和产品信息仅供信息参考。 BenchChem 上可购买的产品专为体外研究设计,这些研究在生物体外进行。体外研究,源自拉丁语 "in glass",涉及在受控实验室环境中使用细胞或组织进行的实验。重要的是要注意,这些产品没有被归类为药物或药品,他们没有得到 FDA 的批准,用于预防、治疗或治愈任何医疗状况、疾病或疾病。我们必须强调,将这些产品以任何形式引入人类或动物的身体都是法律严格禁止的。遵守这些指南对确保研究和实验的法律和道德标准的符合性至关重要。