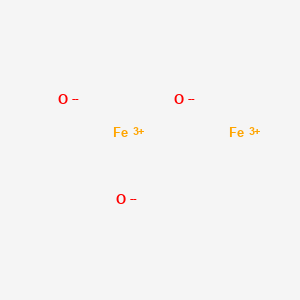
赤铁矿
描述
Hematite, also known as iron(III) oxide, is a heavy and relatively hard oxide mineral with the chemical formula Fe₂O₃. It is one of the most important iron ores due to its high iron content (approximately 70 percent) and its abundance. The name “hematite” is derived from the Greek word for blood, “haima,” due to its red coloration.
科学研究应用
Hematite has a wide range of scientific research applications due to its unique physicochemical properties. Some of the key applications include:
Chemistry: Hematite is used as a catalyst in various chemical reactions, including the synthesis of ammonia and the oxidation of hydrocarbons.
Biology: Hematite nanoparticles are explored for their potential use in biomedical applications, such as drug delivery, magnetic resonance imaging (MRI), and hyperthermia treatment for cancer.
Medicine: Hematite-based materials are investigated for their potential use in biosensors and diagnostic devices.
Industry: Hematite is widely used as a pigment in paints and coatings, as well as a polishing agent for glass and metals
作用机制
Target of Action
Hematite, also known as α-Fe2O3, is a common iron oxide compound . It primarily targets the photoelectrochemical (PEC) water splitting process due to its abundance, stability in an aqueous environment, favorable optical bandgap, and position of the electronic valence band . It also targets the iron (III) reduction process .
Mode of Action
Hematite interacts with its targets through oxidation and reduction reactions. In the PEC water splitting process, hematite acts as a photoanode, absorbing light and generating photo-excited electrons . These electrons are then used to drive the water splitting reaction. In the iron (III) reduction process, hematite facilitates the anaerobic oxidation of ammonia, a process known as Feammox .
Biochemical Pathways
The primary biochemical pathway affected by hematite is the PEC water splitting process. This process involves the conversion of water into hydrogen and oxygen using light energy . Hematite also affects the Feammox process, where ammonia is oxidized under anaerobic conditions with iron (III) acting as the electron acceptor .
Pharmacokinetics
Hematite nanoparticles have been tailored in ellipsoidal and spherical shapes for efficient drug encapsulation and release . These nanoparticles exhibit excellent biocompatibility and have shown potential for enhanced cellular uptake .
Result of Action
The action of hematite results in the splitting of water into hydrogen and oxygen in the PEC process . In the Feammox process, the action of hematite leads to the oxidation of ammonia, resulting in enhanced nitrogen removal . When used in drug delivery systems, hematite nanoparticles can enhance the therapeutic efficacy of drugs .
Action Environment
The action of hematite is influenced by environmental factors such as light and the presence of water. Light is essential for the PEC water splitting process, while water plays a crucial role in both the PEC and Feammox processes . The action of hematite is also influenced by the presence of other substances, such as ammonia in the Feammox process .
生化分析
Biochemical Properties
Hematite nanoparticles have been synthesized using the extract of guava leaves . The biomolecules present in the extract play a crucial role in capping the nanoparticles
Cellular Effects
It has been observed that Hematite nanoparticles exhibit antibacterial activities against both Gram-positive and Gram-negative bacteria . This suggests that Hematite may influence cell function, possibly impacting cell signaling pathways and cellular metabolism.
Molecular Mechanism
It is known that Hematite nanoparticles can interact with various biomolecules
Temporal Effects in Laboratory Settings
In laboratory settings, Hematite nanoparticles have shown enhanced thermal conductivity for base fluids like water and ethylene glycol . This suggests that Hematite may have long-term effects on cellular function. More in vitro or in vivo studies are needed to understand the stability, degradation, and long-term effects of Hematite.
准备方法
Synthetic Routes and Reaction Conditions: Hematite can be synthesized through various methods, including hydrothermal synthesis, sol-gel methods, and precipitation techniques. For instance, hydrothermal synthesis involves mixing iron chloride (FeCl₃) and ammonium hydroxide (NH₄OH) at high temperatures to form hematite nanoparticles. The reaction conditions, such as the concentration of iron ions, the concentration of ammonium hydroxide, and the reaction temperature, significantly influence the particle size and crystallinity of the resulting hematite .
Industrial Production Methods: Industrial production of hematite typically involves the extraction of iron ore from sedimentary deposits. The ore is then subjected to processes such as crushing, grinding, and beneficiation to increase the iron content. The purified ore is subsequently reduced in a blast furnace to produce iron, with hematite serving as a key raw material in this process .
化学反应分析
Types of Reactions: Hematite undergoes various chemical reactions, including reduction, oxidation, and acid leaching. One of the most significant reactions is the reduction of hematite to magnetite (Fe₃O₄) or metallic iron (Fe) in the presence of reducing agents such as carbon monoxide (CO) or hydrogen (H₂). This reaction is crucial in the industrial production of iron .
Common Reagents and Conditions:
Reduction: Hematite is reduced to magnetite or iron using carbon monoxide or hydrogen at high temperatures (500°C to 1000°C).
Oxidation: Hematite can be oxidized to form iron(III) oxide-hydroxide (FeO(OH)) in the presence of oxygen and water.
Acid Leaching: Hematite reacts with acids such as hydrochloric acid (HCl) or sulfuric acid (H₂SO₄) to form soluble iron salts.
Major Products:
Reduction: Magnetite (Fe₃O₄) or metallic iron (Fe).
Oxidation: Iron(III) oxide-hydroxide (FeO(OH)).
Acid Leaching: Soluble iron salts such as iron(III) chloride (FeCl₃) or iron(III) sulfate (Fe₂(SO₄)₃)
相似化合物的比较
Hematite is often compared with other iron oxides, such as magnetite (Fe₃O₄) and maghemite (γ-Fe₂O₃).
Magnetite (Fe₃O₄): Magnetite is another important iron ore with a chemical formula of Fe₃O₄. It is magnetic and has a black or brownish-black color.
Maghemite (γ-Fe₂O₃): Maghemite is a polymorph of hematite with the same chemical formula (Fe₂O₃) but a different crystal structure.
Hematite is unique due to its high iron content, abundance, and diverse forms, making it a versatile material for various applications.
属性
IUPAC Name |
iron(3+);oxygen(2-) | |
---|---|---|
Source | PubChem | |
URL | https://pubchem.ncbi.nlm.nih.gov | |
Description | Data deposited in or computed by PubChem | |
InChI |
InChI=1S/2Fe.3O/q2*+3;3*-2 | |
Source | PubChem | |
URL | https://pubchem.ncbi.nlm.nih.gov | |
Description | Data deposited in or computed by PubChem | |
InChI Key |
LIKBJVNGSGBSGK-UHFFFAOYSA-N | |
Source | PubChem | |
URL | https://pubchem.ncbi.nlm.nih.gov | |
Description | Data deposited in or computed by PubChem | |
Canonical SMILES |
[O-2].[O-2].[O-2].[Fe+3].[Fe+3] | |
Source | PubChem | |
URL | https://pubchem.ncbi.nlm.nih.gov | |
Description | Data deposited in or computed by PubChem | |
Molecular Formula |
Fe2O3 | |
Record name | iron oxide | |
Source | Wikipedia | |
URL | https://en.wikipedia.org/wiki/Iron_oxide | |
Description | Chemical information link to Wikipedia. | |
Source | PubChem | |
URL | https://pubchem.ncbi.nlm.nih.gov | |
Description | Data deposited in or computed by PubChem | |
Molecular Weight |
159.69 g/mol | |
Source | PubChem | |
URL | https://pubchem.ncbi.nlm.nih.gov | |
Description | Data deposited in or computed by PubChem | |
Physical Description |
Dry Powder | |
Record name | Hematite (Fe2O3) | |
Source | EPA Chemicals under the TSCA | |
URL | https://www.epa.gov/chemicals-under-tsca | |
Description | EPA Chemicals under the Toxic Substances Control Act (TSCA) collection contains information on chemicals and their regulations under TSCA, including non-confidential content from the TSCA Chemical Substance Inventory and Chemical Data Reporting. | |
Solubility |
Insol in water; sol in hydrochloric acid, sulfuric acid; slightly sol in nitric acid | |
Record name | HEMATITE | |
Source | Hazardous Substances Data Bank (HSDB) | |
URL | https://pubchem.ncbi.nlm.nih.gov/source/hsdb/2964 | |
Description | The Hazardous Substances Data Bank (HSDB) is a toxicology database that focuses on the toxicology of potentially hazardous chemicals. It provides information on human exposure, industrial hygiene, emergency handling procedures, environmental fate, regulatory requirements, nanomaterials, and related areas. The information in HSDB has been assessed by a Scientific Review Panel. | |
Density |
5.25 | |
Record name | HEMATITE | |
Source | Hazardous Substances Data Bank (HSDB) | |
URL | https://pubchem.ncbi.nlm.nih.gov/source/hsdb/2964 | |
Description | The Hazardous Substances Data Bank (HSDB) is a toxicology database that focuses on the toxicology of potentially hazardous chemicals. It provides information on human exposure, industrial hygiene, emergency handling procedures, environmental fate, regulatory requirements, nanomaterials, and related areas. The information in HSDB has been assessed by a Scientific Review Panel. | |
Color/Form |
Brilliant black to blackish red or brick red mineral, brown to cherry red streak and metallic to dull luster /Hematite, red/, Red-brown hexagonal crystals | |
CAS No. |
1317-60-8, 1309-37-1 | |
Record name | Hematite | |
Source | ChemIDplus | |
URL | https://pubchem.ncbi.nlm.nih.gov/substance/?source=chemidplus&sourceid=0001317608 | |
Description | ChemIDplus is a free, web search system that provides access to the structure and nomenclature authority files used for the identification of chemical substances cited in National Library of Medicine (NLM) databases, including the TOXNET system. | |
Record name | Hematite (Fe2O3) | |
Source | EPA Chemicals under the TSCA | |
URL | https://www.epa.gov/chemicals-under-tsca | |
Description | EPA Chemicals under the Toxic Substances Control Act (TSCA) collection contains information on chemicals and their regulations under TSCA, including non-confidential content from the TSCA Chemical Substance Inventory and Chemical Data Reporting. | |
Record name | Hematite (Fe2O3) | |
Source | European Chemicals Agency (ECHA) | |
URL | https://echa.europa.eu/substance-information/-/substanceinfo/100.013.888 | |
Description | The European Chemicals Agency (ECHA) is an agency of the European Union which is the driving force among regulatory authorities in implementing the EU's groundbreaking chemicals legislation for the benefit of human health and the environment as well as for innovation and competitiveness. | |
Explanation | Use of the information, documents and data from the ECHA website is subject to the terms and conditions of this Legal Notice, and subject to other binding limitations provided for under applicable law, the information, documents and data made available on the ECHA website may be reproduced, distributed and/or used, totally or in part, for non-commercial purposes provided that ECHA is acknowledged as the source: "Source: European Chemicals Agency, http://echa.europa.eu/". Such acknowledgement must be included in each copy of the material. ECHA permits and encourages organisations and individuals to create links to the ECHA website under the following cumulative conditions: Links can only be made to webpages that provide a link to the Legal Notice page. | |
Record name | FERRIC OXIDE RED | |
Source | FDA Global Substance Registration System (GSRS) | |
URL | https://gsrs.ncats.nih.gov/ginas/app/beta/substances/1K09F3G675 | |
Description | The FDA Global Substance Registration System (GSRS) enables the efficient and accurate exchange of information on what substances are in regulated products. Instead of relying on names, which vary across regulatory domains, countries, and regions, the GSRS knowledge base makes it possible for substances to be defined by standardized, scientific descriptions. | |
Explanation | Unless otherwise noted, the contents of the FDA website (www.fda.gov), both text and graphics, are not copyrighted. They are in the public domain and may be republished, reprinted and otherwise used freely by anyone without the need to obtain permission from FDA. Credit to the U.S. Food and Drug Administration as the source is appreciated but not required. | |
Record name | HEMATITE | |
Source | Hazardous Substances Data Bank (HSDB) | |
URL | https://pubchem.ncbi.nlm.nih.gov/source/hsdb/2964 | |
Description | The Hazardous Substances Data Bank (HSDB) is a toxicology database that focuses on the toxicology of potentially hazardous chemicals. It provides information on human exposure, industrial hygiene, emergency handling procedures, environmental fate, regulatory requirements, nanomaterials, and related areas. The information in HSDB has been assessed by a Scientific Review Panel. | |
Melting Point |
1565 °C | |
Record name | HEMATITE | |
Source | Hazardous Substances Data Bank (HSDB) | |
URL | https://pubchem.ncbi.nlm.nih.gov/source/hsdb/2964 | |
Description | The Hazardous Substances Data Bank (HSDB) is a toxicology database that focuses on the toxicology of potentially hazardous chemicals. It provides information on human exposure, industrial hygiene, emergency handling procedures, environmental fate, regulatory requirements, nanomaterials, and related areas. The information in HSDB has been assessed by a Scientific Review Panel. | |
Q1: What is the chemical formula and molecular weight of hematite?
A1: Hematite, also known as α-Fe2O3, has a molecular formula of Fe2O3 and a molecular weight of 159.69 g/mol.
Q2: What spectroscopic techniques are useful for characterizing hematite?
A2: Various spectroscopic techniques prove helpful in characterizing hematite. These include:
- Diffuse Reflectance Spectroscopy (DRS): DRS, particularly its first and second derivative curves of the Kubelka-Munk remission functions, helps identify and quantify hematite and goethite in soil samples. It can differentiate relative content within a profile, reveal the proportions of goethite and hematite, and provide insights into the degree of aluminum substitution. []
- X-ray fluorescence (XRF): This technique is used to quantify the elemental composition of hematite-containing samples, including the content of iron and other elements. []
- Electron Probe Microanalysis (EPMA): This method provides high-resolution elemental analysis of hematite, allowing for the determination of major and trace element concentrations. []
- Micro-Raman Spectroscopy: This technique aids in identifying hematite based on its unique vibrational modes. It can also provide information on the crystallinity and structural disorder within the hematite lattice. []
- Fourier Transform Infrared Spectroscopy (FTIR): FTIR helps identify functional groups and chemical bonds present in hematite. It can also be used to study the adsorption of molecules on the hematite surface. [, ]
- X-ray Photoelectron Spectroscopy (XPS): This surface-sensitive technique can determine the oxidation state of iron in hematite, differentiate between different iron oxide phases, and analyze the chemical composition of surface layers. []
Q3: How does the color of hematite change with temperature?
A3: [] Research shows that the characteristic red color of hematite darkens with increasing temperature, eventually turning black at high temperatures (around 800°C). This change is attributed to changes in the band gap absorption edges of hematite, a semiconductor, as described by the Urbach rule. This temperature-dependent color change has implications for understanding natural phenomena like volcanic eruptions.
Q4: How does particle size influence the spectral properties of hematite?
A4: [] The size of hematite particles significantly affects their spectral properties, particularly in the infrared region. For example, fine-grained red hematite, often found in Martian dust, exhibits different spectral features compared to coarse-grained grey hematite, which is indicative of past water activity.
Q5: What is the role of hematite in contaminant attenuation at mine tailings?
A5: [] Hematite plays a crucial role in immobilizing contaminants in mine tailings, particularly in humid climates. Studies on mine tailings in Zambia and Namibia reveal that secondary hematite formation can effectively sequester contaminants like arsenic, copper, zinc, and lead, reducing their leaching into the surrounding environment.
Q6: How does hematite contribute to the understanding of Martian geology?
A6: [, , , ] The discovery of hematite on Mars, particularly the gray crystalline form in Sinus Meridiani, has significant implications for understanding the planet's geological history and the potential for past liquid water. The presence of hematite spherules, similar to those found in specific terrestrial environments, further strengthens the hypothesis of past aqueous processes on Mars. Analysis of these spherules, combined with insights from their terrestrial counterparts, provides valuable clues about the ancient Martian environment.
Q7: What are the applications of hematite nanoparticles in agriculture?
A7: [] Recent studies have explored the use of hematite nanoparticles as a potential substitute for ferrous sulfate in providing iron to plants. The results indicate that foliar spraying of hematite nanoparticles can be as effective as ferrous sulfate in enhancing wheat yield and protein characteristics. This finding suggests a promising alternative for iron fertilization in agriculture.
Q8: How does aluminum doping affect the morphology of hematite nanoparticles?
A8: [] Aluminum doping plays a crucial role in influencing the morphology of hematite nanoparticles during hydrothermal synthesis. Computational studies, validated by experimental observations, indicate that aluminum preferentially segregates on the (001) surface of hematite. This segregation, coupled with the use of ethanol as a capping agent, promotes the formation of nanoplates with a dominant (001) surface, which exhibits high activity in various catalytic applications.
Q9: How can the photoelectrochemical performance of hematite be enhanced for solar water splitting?
A9: Several strategies can be employed to enhance the photoelectrochemical performance of hematite for solar water splitting:
- Oxygen-Deficient Atmosphere Treatment: Annealing hematite films in an oxygen-deficient atmosphere during synthesis can significantly enhance their photoelectrochemical performance. [] This treatment improves the catalytic activity of hematite for water oxidation, leading to a more efficient hematite-electrolyte interface.
- Foreign In3+ Ion Treatment: Treating hematite nanosheets with In3+ ions during solvothermal synthesis can significantly boost their photoelectrochemical properties for water splitting. [] While In3+ ions do not dope into the hematite lattice, this treatment increases active surface area, promotes higher light absorption, and enhances charge carrier density and separation efficiency.
- TiO2 Surface Treatment: Coating hematite nanorods with a thin layer of TiO2 using atomic layer deposition (ALD) and subsequent annealing at high temperatures significantly improves their photoelectrochemical water oxidation performance. [] This enhancement is attributed to increased surface states at the hematite surface, leading to better charge separation, reduced electron-hole recombination, and improved photocurrent.
Q10: What is a key challenge in using hematite for electrolytic iron production and how is it being addressed?
A10: [] A major challenge in electrolytic iron production using hematite is the poorly understood adsorption process of hematite particles onto the steel electrode. Researchers are developing quantitative methods to measure this adsorption, aiming to optimize the process and improve the efficiency of iron production.
体外研究产品的免责声明和信息
请注意,BenchChem 上展示的所有文章和产品信息仅供信息参考。 BenchChem 上可购买的产品专为体外研究设计,这些研究在生物体外进行。体外研究,源自拉丁语 "in glass",涉及在受控实验室环境中使用细胞或组织进行的实验。重要的是要注意,这些产品没有被归类为药物或药品,他们没有得到 FDA 的批准,用于预防、治疗或治愈任何医疗状况、疾病或疾病。我们必须强调,将这些产品以任何形式引入人类或动物的身体都是法律严格禁止的。遵守这些指南对确保研究和实验的法律和道德标准的符合性至关重要。