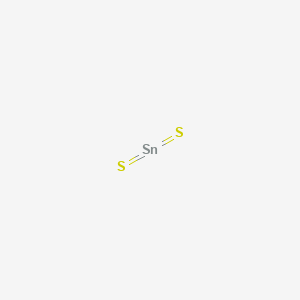
硫化锡 (SnS2)
描述
Tin (IV) sulfide is a compound with the formula SnS2. It crystallizes in the cadmium iodide motif, with the Sn (IV) situated in "octahedral holes’ defined by six sulfide centers . It occurs naturally as the rare mineral berndtite . It is useful as a semiconductor material with a bandgap of 2.2 eV .
Synthesis Analysis
Tin Sulfide (SnS) nanoparticles are synthesized with three different solvents through the hydrothermal method . The prepared SnS nanoparticles exist in the orthorhombic phase . The SEM analysis clearly picturizes the morphological changes in the SnS nanoparticles synthesized through different solvents .Molecular Structure Analysis
SnS2 crystallizes in the form of an orthorhombic structure, where each Sn (II) atom is coordinated to six S atoms - with three short Sn–S bonds within the surface and three longer Sn-S bonds connecting the outer surface of the same layer .Chemical Reactions Analysis
Tin (IV) Oxide reacts with Sulfur Dioxide to form Stannic Sulfide and Dioxygen . Also, Tin (IV) Oxide reacts with Hydrogen Sulfide to form Stannic Sulfide and Water .Physical And Chemical Properties Analysis
Tin (IV) sulfide is a gold-colored or brown solid . It has a bandgap of 2.2 eV . The optical studies revealed that the band gap between 2.65 and 2.72 eV and high optical transmittance 98% .科学研究应用
Solar Energy Conversion
Tin (II) sulfide (SnS) is an attractive semiconductor for solar energy conversion in thin film devices due to its bandgap of around 1.3 eV in its orthorhombic polymorph, and a band gap energy of 1.5–1.7 eV for the cubic polymorph . These properties are commensurate with efficient light harvesting, combined with a high absorption coefficient across the NIR–visible region of the electromagnetic spectrum .
Optoelectronic Devices
SnS applications in optoelectronic devices include photovoltaics and photodetectors . The bandgap energy can be tuned by thinning the material to nanoscale dimensions, enabling a variety of future applications .
Energy Storage
Tin sulfide has been investigated for use in lithium- and sodium-ion batteries . Its unique properties make it a promising material for energy storage applications .
Sensors
Tin sulfide can be used in sensor applications . Its unique properties, such as its high natural abundance and a relative lack of toxicity, make it a sustainable and accessible material for sensor applications .
Two-Dimensional Electronic Devices
Tin sulfides are investigated for applications in two-dimensional electronic devices . Their unique optical, electronic, and mechanical properties open up windows for a broad range of promising applications .
Photocatalysts
Tin sulfides have been explored as photocatalysts . Their unique properties make them suitable for a wide variety of photocatalytic applications .
作用机制
Target of Action
Tin sulfide (SnS2) is a compound that primarily targets the lattice dynamics of various materials . It has a layered bonding structure, similar to that of 2D transition-metal dichalcogenides such as MoS2 . The compound crystallizes in the cadmium iodide motif, with the Sn (IV) situated in “octahedral holes” defined by six sulfide centers .
Mode of Action
The interaction of SnS2 with its targets involves the manipulation of the harmonic phonon dispersion and vibrational density of states . This interaction reveals phonon bandgaps between low- and high-frequency modes consisting of Sn and S motion, respectively . It also evidences a bond-strength hierarchy in the low-dimensional SnS2 crystals .
Biochemical Pathways
The primary biochemical pathway affected by SnS2 is the thermal transport pathway . The spectral linewidths of SnS2 are used to model the thermal transport, and the calculations indicate that SnS2 has a very low lattice thermal conductivity . This property potentially gives it superior performance to SnS as a candidate thermoelectric material .
Result of Action
The molecular and cellular effects of SnS2’s action primarily involve changes in the thermal and electrical properties of the target material . For instance, SnS2 has been shown to have a high optical absorption coefficient and a bandgap close to the theoretical optimum for peak photovoltaic efficiency . These properties make it suitable for use in various applications, including as a buffer layer in photovoltaic devices, a high surface-area photocatalyst, and a photodetector .
Action Environment
Environmental factors can significantly influence the action, efficacy, and stability of SnS2. For instance, the formation of the SnS2 phase is favorable at higher temperatures . Moreover, the SnS2 phase depends on both the temperature and the H2S/SnCl4 concentration ratio . These factors highlight the importance of carefully controlled environmental conditions in the synthesis and application of SnS2.
安全和危害
未来方向
属性
IUPAC Name |
bis(sulfanylidene)tin | |
---|---|---|
Source | PubChem | |
URL | https://pubchem.ncbi.nlm.nih.gov | |
Description | Data deposited in or computed by PubChem | |
InChI |
InChI=1S/2S.Sn | |
Source | PubChem | |
URL | https://pubchem.ncbi.nlm.nih.gov | |
Description | Data deposited in or computed by PubChem | |
InChI Key |
ALRFTTOJSPMYSY-UHFFFAOYSA-N | |
Source | PubChem | |
URL | https://pubchem.ncbi.nlm.nih.gov | |
Description | Data deposited in or computed by PubChem | |
Canonical SMILES |
S=[Sn]=S | |
Source | PubChem | |
URL | https://pubchem.ncbi.nlm.nih.gov | |
Description | Data deposited in or computed by PubChem | |
Molecular Formula |
SnS2, S2Sn | |
Record name | tin(IV) sulfide | |
Source | Wikipedia | |
URL | https://en.wikipedia.org/wiki/Tin(IV)_sulfide | |
Description | Chemical information link to Wikipedia. | |
Source | PubChem | |
URL | https://pubchem.ncbi.nlm.nih.gov | |
Description | Data deposited in or computed by PubChem | |
Molecular Weight |
182.8 g/mol | |
Source | PubChem | |
URL | https://pubchem.ncbi.nlm.nih.gov | |
Description | Data deposited in or computed by PubChem | |
Physical Description |
Golden solid with a metallic luster; [Merck Index] Yellow or gold powder; [Alfa Aesar MSDS] | |
Record name | Stannic sulfide | |
Source | Haz-Map, Information on Hazardous Chemicals and Occupational Diseases | |
URL | https://haz-map.com/Agents/17710 | |
Description | Haz-Map® is an occupational health database designed for health and safety professionals and for consumers seeking information about the adverse effects of workplace exposures to chemical and biological agents. | |
Explanation | Copyright (c) 2022 Haz-Map(R). All rights reserved. Unless otherwise indicated, all materials from Haz-Map are copyrighted by Haz-Map(R). No part of these materials, either text or image may be used for any purpose other than for personal use. Therefore, reproduction, modification, storage in a retrieval system or retransmission, in any form or by any means, electronic, mechanical or otherwise, for reasons other than personal use, is strictly prohibited without prior written permission. | |
Product Name |
Tin sulfide (SnS2) | |
CAS RN |
1315-01-1 | |
Record name | Tin sulfide (SnS2) | |
Source | CAS Common Chemistry | |
URL | https://commonchemistry.cas.org/detail?cas_rn=1315-01-1 | |
Description | CAS Common Chemistry is an open community resource for accessing chemical information. Nearly 500,000 chemical substances from CAS REGISTRY cover areas of community interest, including common and frequently regulated chemicals, and those relevant to high school and undergraduate chemistry classes. This chemical information, curated by our expert scientists, is provided in alignment with our mission as a division of the American Chemical Society. | |
Explanation | The data from CAS Common Chemistry is provided under a CC-BY-NC 4.0 license, unless otherwise stated. | |
Record name | Tin sulfide (SnS2) | |
Source | EPA Chemicals under the TSCA | |
URL | https://www.epa.gov/chemicals-under-tsca | |
Description | EPA Chemicals under the Toxic Substances Control Act (TSCA) collection contains information on chemicals and their regulations under TSCA, including non-confidential content from the TSCA Chemical Substance Inventory and Chemical Data Reporting. | |
体外研究产品的免责声明和信息
请注意,BenchChem 上展示的所有文章和产品信息仅供信息参考。 BenchChem 上可购买的产品专为体外研究设计,这些研究在生物体外进行。体外研究,源自拉丁语 "in glass",涉及在受控实验室环境中使用细胞或组织进行的实验。重要的是要注意,这些产品没有被归类为药物或药品,他们没有得到 FDA 的批准,用于预防、治疗或治愈任何医疗状况、疾病或疾病。我们必须强调,将这些产品以任何形式引入人类或动物的身体都是法律严格禁止的。遵守这些指南对确保研究和实验的法律和道德标准的符合性至关重要。