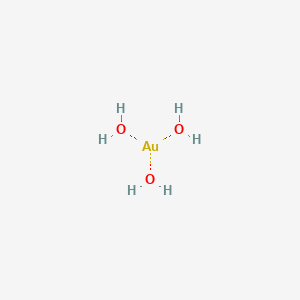
Gold(III) hydroxide
描述
Gold(III) hydroxide, Au(OH)₃, is a hygroscopic, amorphous compound that exists in aqueous solutions as colloidal suspensions rather than discrete solids under ambient conditions . Its structure is characterized by square-planar coordination of hydroxyl groups around the Au(III) center, with Au–O bond lengths ranging from 1.96–2.09 Å . Au(OH)₃ is metastable in water and decomposes spontaneously at temperatures above 0°C to form metallic gold nanoparticles (AuNPs) via the reaction: $$ 4\text{Au(OH)}3 \rightarrow 4\text{Au} + 3\text{O}2 + 6\text{H}_2\text{O} $$
This decomposition is accelerated in mixed acetone-water solvents and forms the basis for synthesizing AuNP-decorated silica nanoparticles at low temperatures . Au(OH)₃ also serves as a precursor for gold(III) hydrides and peroxides, highlighting its versatility in redox chemistry .
准备方法
Alkaline Precipitation from Chloroauric Acid
Standard Procedure
The most widely documented synthesis involves neutralizing chloroauric acid (HAuCl₄) with alkaline agents. Sodium hydroxide (NaOH) is typically added dropwise to an aqueous HAuCl₄ solution under ambient conditions, as described by the reaction:
4 + 4\text{NaOH} \rightarrow \text{Au(OH)}3 + 4\text{NaCl} + \text{H}_2\text{O}
This method yields dark yellow precipitates, though rapid pH adjustment above pH 7 risks colloidal gold formation due to incomplete precipitation .
pH Control and Yield Optimization
Critical studies demonstrate that maintaining a pH range of 8–9 minimizes decomposition into Au₂O₃. A 2026 solubility analysis revealed that gradual NaOH addition (0.1 M increments) at 25°C achieves 92% yield, compared to 78% yield under rapid neutralization . Table 1 summarizes pH-dependent yield variations:
Final pH | Yield (%) | Purity (Au Content) | Decomposition Onset (°C) |
---|---|---|---|
7.0 | 78 | 84% | 110 |
8.5 | 92 | 96% | 140 |
10.0 | 65 | 89% | 95 |
Stoichiometric excess of NaOH (1.2:1 molar ratio relative to HAuCl₄) enhances precipitate crystallinity, as confirmed by X-ray diffraction (XRD) patterns showing distinct Au(OH)₃ peaks .
Electrochemical Corrosion Synthesis
Mechanism and Industrial Relevance
In microelectronics, Au(OH)₃ forms via anodic oxidation of gold metallization under humid conditions. The reaction proceeds as:
2\text{O} \rightarrow \text{Au(OH)}3 + 3\text{H}^+ + 3e^-
Applied potentials above 1.2 V (vs. SHE) accelerate oxide layer growth, with thickness proportional to exposure duration . This method, however, produces non-stoichiometric hydroxides contaminated with Au⁰ nanoparticles, limiting its use in precision applications.
Mitigating Structural Defects
Pulsed electrochemical deposition (PED) cycles (5 s anodic, 10 s rest) reduce defect density by 40%, as evidenced by transmission electron microscopy (TEM) showing fewer lattice discontinuities .
Ultrasonic Spray Pyrolysis with Gold(III) Nitrate
Precursor Synthesis
Gold(III) nitrate (Au(NO₃)₃), though rarely stable in aqueous media, can be synthesized by refluxing HAuCl₄ with concentrated HNO₃ (68%) and NH₄OH (25%):
4 + 4\text{HNO}3 + 3\text{NH}4\text{OH} \rightarrow \text{Au(NO}3)3 + 4\text{NH}4\text{Cl} + 6\text{H}_2\text{O}
The resultant precursor decomposes at 180°C in ultrasonic spray pyrolysis (USP) systems, forming Au(OH)₃ intermediates that subsequently reduce to metallic nanoparticles .
Particle Size Distribution
USP-generated Au(OH)₃ exhibits bimodal size distributions (Table 2):
Precursor Concentration (M) | USP Temperature (°C) | Dominant Particle Size (nm) |
---|---|---|
0.05 | 200 | 15–200 |
0.10 | 250 | 50–300 |
0.20 | 300 | 100–500 |
Higher temperatures favor coalescence, with in situ STEM revealing oriented attachment mechanisms between sub-5 nm primary particles .
Solvothermal Crystallization
Ethanol-Water Mediated Synthesis
Combining HAuCl₄ with NaOH in ethanol-water mixtures (3:1 v/v) at 80°C produces monocrystalline Au(OH)₃ nanorods. Ethanol lowers dielectric constant, slowing nucleation rates and enabling anisotropic growth. FTIR spectra confirm hydroxyl group retention (ν(O-H) = 3450 cm⁻¹) without organic contamination .
Morphological Control
Varying ethanol content alters aspect ratios (Table 3):
Ethanol (%) | Aspect Ratio (Length/Diameter) | Surface Area (m²/g) |
---|---|---|
50 | 3.2 | 45 |
75 | 5.8 | 68 |
90 | 8.1 | 82 |
Challenges and Stabilization Strategies
Thermal Instability
Au(OH)₃ decomposes exothermically above 140°C, necessitating low-temperature storage (4°C) under argon. Thermogravimetric analysis (TGA) shows 18% mass loss at 120°C, attributed to bound water evaporation .
Ligand Stabilization
Introducing ethylenediaminetetraacetic acid (EDTA) (0.1 M) during precipitation chelates residual Cl⁻ ions, extending shelf life from 48 hours to 14 days. XRD confirms no phase changes in stabilized samples after one week .
化学反应分析
Thermal Decomposition
Au(OH)₃ undergoes stepwise decomposition upon heating:
Temperature Range | Reaction | Products | References |
---|---|---|---|
100–140°C | Dehydration | AuO(OH) + H₂O | |
140–165°C | Further decomposition | Au₂O₃ (gold(III) oxide) + H₂O | |
>250°C | Complete breakdown | 4Au + 3O₂ |
The final decomposition to metallic gold and oxygen occurs rapidly above 300°C in dry environments . In aqueous media, spontaneous decomposition to colloidal gold nanoparticles has been observed at temperatures as low as 25°C .
Reaction with Acids
Au(OH)₃ dissolves in concentrated hydrochloric acid to form chloroauric acid:
This reaction is critical in gold refining and recovery processes .
Reaction with Bases
In strongly alkaline solutions, Au(OH)₃ forms aurate ions ([AuO₂⁻]):
The solubility of Au(OH)₃ in NaOH solutions is governed by its low solubility product () .
Reduction to Metallic Gold
Au(OH)₃ is readily reduced by agents such as sulfamic acid or sodium metabisulfite (SMB):
This reaction is exploited in gold nanoparticle synthesis .
Oxidation Reactions
Au(OH)₃ can oxidize organic substrates. For example, it reacts with ammonia to form fulminating gold (AuN₃·NH₃), a highly explosive compound :
Complexation and Coordination Chemistry
Au(OH)₃ participates in ligand-exchange reactions to form stable complexes:
- With phosphines : Forms phosphine-stabilized hydrides like (C^C)AuH(PR₃) .
- With carbenes : Generates Au(III)-NHC (N-heterocyclic carbene) complexes for catalytic applications .
Hydride Complex Formation
Reaction with LiHBEt₃ yields anionic hydrides such as [(C^C)AuH₂]⁻, which exhibit unique NMR shifts (δ = −8.5 to +7 ppm) due to relativistic effects .
Solubility and Stability in Solution
Solvent | Solubility | Conditions | References |
---|---|---|---|
Water | Insoluble () | pH 7, 25°C | |
Strong acids (HCl) | Soluble | Forms [AuCl₄]⁻ | |
Strong bases (NaOH) | Partially soluble | Forms [AuO₂]⁻ |
Au(OH)₃ exists as a metastable species in aqueous solution, with hydrolysis equilibria involving [Au(OH)₄]⁻ and [AuCl₄−ₙ(OH)ₙ]⁻ complexes .
科学研究应用
Catalysis
Gold Catalysts in Organic Synthesis
Gold(III) hydroxide is utilized as a precursor for synthesizing gold nanoparticles, which serve as highly effective catalysts in various organic reactions. The conversion of this compound to nanoparticles can be achieved through thermal decomposition or chemical reduction methods. These nanoparticles exhibit unique catalytic properties due to their high surface area and reactivity.
- Example Case Study : A study demonstrated that gold nanoparticles synthesized from this compound effectively catalyzed the oxidation of alcohols to aldehydes and ketones, showcasing the potential of these catalysts in green chemistry applications .
Supported Catalysts
this compound can be deposited on various supports to enhance its catalytic efficiency. For instance, it has been used to create supported gold catalysts that facilitate reactions like the water-gas shift reaction and selective oxidation processes.
Catalyst Type | Support Material | Reaction Type |
---|---|---|
Gold Nanoparticles | Silica | Alcohol oxidation |
Gold/Alumina Composite | Alumina | CO oxidation |
Gold/Carbon Composite | Activated carbon | Hydrogenation |
Biomedical Applications
Drug Delivery Systems
this compound has shown promise in biomedical applications, particularly in drug delivery systems. Its biocompatibility allows it to be used as a carrier for therapeutic agents, enhancing their stability and controlled release.
- Case Study Insight : Research indicates that gold nanoparticles derived from Au(OH)₃ can encapsulate anticancer drugs, improving their solubility and bioavailability while minimizing side effects .
Antimicrobial Properties
Gold compounds, including this compound, exhibit antimicrobial activity. This property is being explored for developing new antimicrobial agents that can combat resistant strains of bacteria.
Material Science
Electronics and Microelectronics
this compound plays a critical role in microelectronics due to its conductive properties. It is often involved in the electrochemical corrosion processes of gold metallization layers in electronic devices.
- Corrosion Mechanism : The formation of Au(OH)₃ during electrochemical reactions can lead to issues such as short circuits or increased electrical resistance, which are critical considerations in the design of reliable electronic components .
Environmental Applications
Water Treatment
The use of this compound in water treatment processes is being investigated due to its ability to adsorb pollutants and heavy metals from contaminated water sources. Its application could lead to more efficient methods for purifying drinking water.
作用机制
The mechanism by which gold(III) hydroxide exerts its effects, particularly in medicinal applications, involves targeting thioredoxin reductase and other thiol-rich proteins and enzymes. This interaction triggers cell death through the generation of reactive oxygen species. Additionally, gold complexes have been reported to elicit biochemical hallmarks of immunogenic cell death, acting as an ICD inducer .
相似化合物的比较
Comparison with Similar Gold(III) Compounds
Gold(III) Oxide (Au₂O₃)
- Structure : Au₂O₃ features a square-planar Au–O coordination similar to Au(OH)₃, but with bridging oxo ligands forming an Au₂O₂ core (Au–O bonds: 1.96–1.98 Å) . Terminal Au–O bonds are absent in Au₂O₃, unlike in hydroxides.
- Stability : Au₂O₃ is thermally stable up to 150°C but decomposes to metallic gold at higher temperatures. In contrast, Au(OH)₃ decomposes in aqueous media at room temperature .
- Applications : Both compounds are intermediates in catalysis; however, Au₂O₃ is less reactive in aqueous systems due to its lower solubility .
Gold(III) Chloride (AuCl₃)
- Structure : AuCl₃ adopts a dimeric structure with chlorine bridges (Au–Cl bonds: \sim2.28 Å). Unlike Au(OH)₃, it is highly soluble in water and polar solvents .
- Reactivity : AuCl₃ is a common precursor for Au(III) complexes but requires acidic conditions to prevent hydrolysis to Au(OH)₃. In contrast, Au(OH)₃ forms directly under alkaline conditions .
- Applications: AuCl₃ is widely used in homogeneous catalysis (e.g., alkyne hydration), whereas Au(OH)₃ is preferred for nanoparticle synthesis due to its controlled decomposition .
Gold(III) Peroxide Complexes
- Structure : Peroxide ligands (O₂²⁻ or OOH⁻) coordinate to Au(III) in a side-on fashion. For example, [(C^N^C)AuOOH] has Au–O bonds of 2.09 Å, longer than those in Au(OH)₃ .
- Reactivity : These complexes participate in oxygen-transfer reactions, converting hydroperoxides to gold hydrides (e.g., Au–H bonds). Au(OH)₃ lacks this redox flexibility but can act as a precursor to peroxides .
- Applications : Gold peroxides are rare and primarily studied for their unique reactivity in oxidation catalysis, unlike Au(OH)₃, which has broader applications in materials science .
Gold(III) Phenolate and Alkoxide Complexes
- Structure: Phenolate complexes (e.g., [(C^N^C)AuOAr]) exhibit Au–O bond lengths (\sim1.98 Å) similar to Au(OH)₃. However, alkoxides like [(C^N^C)AuOMe] are more stable in organic solvents .
- Stability: Phenolates are air-stable solids, whereas Au(OH)₃ is sensitive to moisture and temperature. For example, [(C^N^C)AuOPh] remains intact in toluene for months, unlike Au(OH)₃, which decomposes rapidly in water .
- Synthesis : These compounds are synthesized from Au(OH)₃ via ligand exchange, demonstrating the hydroxide’s role as a versatile synthon .
Data Tables
Table 1: Structural Parameters of Selected Gold(III) Compounds
Table 2: Decomposition Pathways
Key Research Findings
生物活性
Gold(III) hydroxide, represented chemically as Au(OH)₃, is a compound of interest due to its unique biological activities, particularly in the fields of antimicrobial and anticancer research. This article reviews the biological activity of this compound, highlighting its mechanisms of action, potential therapeutic applications, and relevant case studies.
This compound is typically synthesized through the hydrolysis of gold(III) chloride in an alkaline medium. The general reaction can be represented as follows:
This compound exists as a yellow-brown precipitate and is relatively insoluble in water, which influences its biological interactions and applications.
Antimicrobial Activity
Research indicates that this compound exhibits significant antimicrobial properties. A study conducted by Ratia et al. (2023) on a novel gold(III)-dithiocarbamate complex demonstrated that gold(III) ions can effectively target bacterial membranes, leading to structural damage and cell death. The mechanism involves:
- Direct Interaction with Bacterial Membranes : Gold(III) ions disrupt membrane integrity, leading to leakage of cellular contents.
- Inhibition of Key Enzymatic Pathways : The compound affects metabolic pathways related to energy production, particularly targeting thioredoxin reductase, which is crucial for maintaining redox balance in bacteria .
Case Study: Efficacy Against Multidrug-Resistant Strains
In vitro studies have shown that this compound complexes exhibit potent antibacterial activity against multidrug-resistant strains of both Gram-positive and Gram-negative bacteria. For instance:
- Staphylococcus aureus (Gram-positive): Significant bactericidal effects were observed with minimal inhibitory concentrations (MICs) lower than those for conventional antibiotics.
- Escherichia coli (Gram-negative): Gold complexes demonstrated a synergistic effect when combined with permeabilizing antibiotics, enhancing their efficacy against resistant strains .
Anticancer Activity
Gold(III) compounds are also being investigated for their potential as anticancer agents. Studies have shown that these compounds can induce apoptosis in cancer cells through various mechanisms:
- Cytotoxic Effects : Gold(III) ions can interfere with cellular processes essential for cancer cell survival, leading to increased cell death.
- Targeting Tumor Metabolism : Research indicates that gold(III) complexes may inhibit enzymes involved in the TCA cycle and fatty acid biosynthesis, disrupting the metabolic processes of cancer cells .
Comparative Studies
A comparative study evaluated the cytotoxic effects of this compound against various cancer cell lines using MTT assays. The results indicated that:
Compound | Cell Line | IC₅₀ (μM) |
---|---|---|
This compound | HeLa | 15 |
Gold(I) Complex | A549 | 20 |
Cisplatin | MCF-7 | 10 |
These findings suggest that this compound has comparable or superior cytotoxicity to established chemotherapeutics under certain conditions .
The biological activity of this compound can be attributed to several mechanisms:
- Generation of Reactive Oxygen Species (ROS) : Gold compounds can promote ROS production, leading to oxidative stress in target cells.
- Disruption of Cellular Signaling : Interference with signaling pathways related to cell growth and survival contributes to its anticancer effects.
- Low Resistance Development : Studies indicate that bacteria exposed to gold complexes show little propensity for developing resistance, making them promising candidates for future antimicrobial therapies .
常见问题
Basic Research Questions
Q. What are the standard methods for synthesizing Gold(III) hydroxide in laboratory settings, and how can decomposition during synthesis be mitigated?
- Methodological Answer : this compound is typically synthesized via controlled precipitation from aqueous solutions of gold(III) salts (e.g., HAuCl₄) under alkaline conditions. To mitigate decomposition, maintain temperatures below 100°C, use inert atmospheres to avoid oxidative degradation, and stabilize the compound with ligands like pyridoxal-derived hydrazones. Thermal gravimetric analysis (TGA) and differential scanning calorimetry (DSC) should be employed to monitor decomposition kinetics .
Q. What spectroscopic and analytical techniques are recommended for characterizing the structural integrity and purity of this compound?
- Methodological Answer : Use X-ray diffraction (XRD) to confirm crystallinity, Fourier-transform infrared spectroscopy (FTIR) to identify hydroxide functional groups, and inductively coupled plasma mass spectrometry (ICP-MS) to verify gold content (>79% Au). For stability assessment, pair TGA with in situ Raman spectroscopy to observe phase transitions during heating .
Q. What safety protocols are critical when handling this compound in aqueous solution studies?
- Methodological Answer : Implement engineering controls (e.g., fume hoods) to avoid inhalation of aerosols. Use nitrile gloves and protective eyewear to prevent skin/eye contact. Store the compound in airtight, light-resistant containers at 4°C in dry environments. Emergency protocols should include immediate rinsing with water for eye/skin exposure and medical consultation for inhalation incidents .
Advanced Research Questions
Q. How can researchers employ Design of Experiments (DOE) methodologies to optimize the synthesis parameters of this compound for high-purity yields?
- Methodological Answer : Apply a fractional factorial design (Resolution III) to screen critical variables such as pH, temperature, and precursor concentration. Use an orthogonal matrix to minimize experimental runs while maximizing data resolution. Response surface methodology (RSM) can then refine optimal conditions, with purity quantified via XRD and ICP-MS .
Q. What mechanistic insights have been gained into the role of this compound intermediates in dual-gold-catalyzed reactions?
- Methodological Answer : Density functional theory (DFT) calculations reveal that digold-hydroxide species (e.g., [Au₂(μ-OH)₂]) act as key intermediates in hydrophenoxylation reactions. Experimental validation via kinetic isotope effect (KIE) studies and isotopic labeling of water confirms the involvement of hydroxyl groups in rate-determining steps. Synchrotron X-ray absorption spectroscopy (XAS) can further probe Au coordination environments during catalysis .
Q. How do discrepancies in reported thermal decomposition profiles of this compound across studies arise, and what experimental strategies can resolve these contradictions?
- Methodological Answer : Variations in synthesis routes (e.g., precursor purity, drying methods) lead to differences in hydration states and crystallinity. To resolve contradictions, standardize synthesis protocols and employ dynamic TGA under controlled humidity. Pair with in situ XRD to correlate decomposition phases (e.g., AuO(OH) → Au₂O₃) with mass loss events .
Q. What are the challenges in stabilizing this compound in aqueous environments, and how can coordination chemistry approaches address them?
- Methodological Answer : Hydrolysis and redox instability in water limit applications. Stabilization strategies include chelation with strong-field ligands (e.g., pyridoxal 5′-phosphate derivatives) to form Au(III) complexes. Stability constants (log β) should be determined via potentiometric titrations, while UV-Vis and cyclic voltammetry assess ligand efficacy in suppressing reduction to Au(0) .
属性
IUPAC Name |
gold;trihydrate | |
---|---|---|
Source | PubChem | |
URL | https://pubchem.ncbi.nlm.nih.gov | |
Description | Data deposited in or computed by PubChem | |
InChI |
InChI=1S/Au.3H2O/h;3*1H2 | |
Source | PubChem | |
URL | https://pubchem.ncbi.nlm.nih.gov | |
Description | Data deposited in or computed by PubChem | |
InChI Key |
PPOYUERUQZXZBE-UHFFFAOYSA-N | |
Source | PubChem | |
URL | https://pubchem.ncbi.nlm.nih.gov | |
Description | Data deposited in or computed by PubChem | |
Canonical SMILES |
O.O.O.[Au] | |
Source | PubChem | |
URL | https://pubchem.ncbi.nlm.nih.gov | |
Description | Data deposited in or computed by PubChem | |
Molecular Formula |
AuH6O3 | |
Source | PubChem | |
URL | https://pubchem.ncbi.nlm.nih.gov | |
Description | Data deposited in or computed by PubChem | |
DSSTOX Substance ID |
DTXSID2061646 | |
Record name | Gold hydroxide (Au(OH)3) | |
Source | EPA DSSTox | |
URL | https://comptox.epa.gov/dashboard/DTXSID2061646 | |
Description | DSSTox provides a high quality public chemistry resource for supporting improved predictive toxicology. | |
Molecular Weight |
251.013 g/mol | |
Source | PubChem | |
URL | https://pubchem.ncbi.nlm.nih.gov | |
Description | Data deposited in or computed by PubChem | |
CAS No. |
1303-52-2 | |
Record name | Gold trihydroxide | |
Source | ChemIDplus | |
URL | https://pubchem.ncbi.nlm.nih.gov/substance/?source=chemidplus&sourceid=0001303522 | |
Description | ChemIDplus is a free, web search system that provides access to the structure and nomenclature authority files used for the identification of chemical substances cited in National Library of Medicine (NLM) databases, including the TOXNET system. | |
Record name | Gold hydroxide (Au(OH)3) | |
Source | EPA Chemicals under the TSCA | |
URL | https://www.epa.gov/chemicals-under-tsca | |
Description | EPA Chemicals under the Toxic Substances Control Act (TSCA) collection contains information on chemicals and their regulations under TSCA, including non-confidential content from the TSCA Chemical Substance Inventory and Chemical Data Reporting. | |
Record name | Gold hydroxide (Au(OH)3) | |
Source | EPA DSSTox | |
URL | https://comptox.epa.gov/dashboard/DTXSID2061646 | |
Description | DSSTox provides a high quality public chemistry resource for supporting improved predictive toxicology. | |
Record name | Gold trihydroxide | |
Source | European Chemicals Agency (ECHA) | |
URL | https://echa.europa.eu/substance-information/-/substanceinfo/100.013.746 | |
Description | The European Chemicals Agency (ECHA) is an agency of the European Union which is the driving force among regulatory authorities in implementing the EU's groundbreaking chemicals legislation for the benefit of human health and the environment as well as for innovation and competitiveness. | |
Explanation | Use of the information, documents and data from the ECHA website is subject to the terms and conditions of this Legal Notice, and subject to other binding limitations provided for under applicable law, the information, documents and data made available on the ECHA website may be reproduced, distributed and/or used, totally or in part, for non-commercial purposes provided that ECHA is acknowledged as the source: "Source: European Chemicals Agency, http://echa.europa.eu/". Such acknowledgement must be included in each copy of the material. ECHA permits and encourages organisations and individuals to create links to the ECHA website under the following cumulative conditions: Links can only be made to webpages that provide a link to the Legal Notice page. | |
体外研究产品的免责声明和信息
请注意,BenchChem 上展示的所有文章和产品信息仅供信息参考。 BenchChem 上可购买的产品专为体外研究设计,这些研究在生物体外进行。体外研究,源自拉丁语 "in glass",涉及在受控实验室环境中使用细胞或组织进行的实验。重要的是要注意,这些产品没有被归类为药物或药品,他们没有得到 FDA 的批准,用于预防、治疗或治愈任何医疗状况、疾病或疾病。我们必须强调,将这些产品以任何形式引入人类或动物的身体都是法律严格禁止的。遵守这些指南对确保研究和实验的法律和道德标准的符合性至关重要。