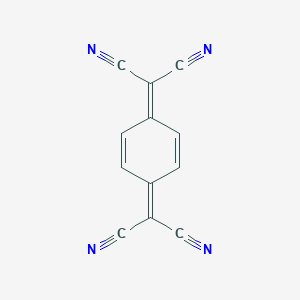
7,7,8,8-四氰基对苯醌二甲烷
概述
描述
7,7,8,8-Tetracyanoquinodimethane is an organic compound with the chemical formula (N≡C−)2C=C6H4=C(−C≡N)2. It is an orange crystalline solid and a relative of para-quinone. This cyanocarbon is a prototypical electron acceptor, widely used to prepare charge transfer salts, which are of significant interest in molecular electronics .
科学研究应用
7,7,8,8-Tetracyanoquinodimethane has diverse applications in scientific research, including:
Molecular Electronics:
Organic Semiconductors:
CO2 Separation:
作用机制
Target of Action:
TCNQ is a prototypical electron acceptor that forms charge-transfer (CT) complexes with various electron-donor molecules and metal ions . Its primary targets include these electron donors, which interact with TCNQ to create CT complexes.
Mode of Action:
The interaction between TCNQ and its targets involves electron transfer. When TCNQ accepts electrons from donors, it forms radical anions (TCNQ−) and undergoes reversible redox reactions . This process leads to the breakdown of TCNQ dimers, resulting in massive delocalized electrons.
Biochemical Pathways:
TCNQ-based complexes impact several pathways. For instance, TCNQ–tetrathiafulvalene (TTF) organic salts exhibit high electrical conductivity. These materials have been studied for applications in electron transport and organic magnets .
Action Environment:
Environmental factors play a crucial role in TCNQ’s efficacy and stability. Temperature, humidity, and exposure to light affect its behavior. For instance, TCNQ-based materials are sensitive to moisture and oxygen.
: New aspect of photophysics of 7,7,8,8-tetracyanoquinodimethane and its solvated complexes : Real-time monitoring of the lithiation process in organic materials
生化分析
Biochemical Properties
It is known that this compound is a strong electron acceptor, forming charge transferring chains and ion radical salts . These properties suggest that 7,7,8,8-Tetracyanoquinodimethane could potentially interact with various enzymes, proteins, and other biomolecules in a biochemical context, although specific interactions have not been reported.
Molecular Mechanism
It is known to act as an electron acceptor, suggesting that it could potentially exert its effects at the molecular level through binding interactions with biomolecules, enzyme inhibition or activation, and changes in gene expression
准备方法
7,7,8,8-Tetracyanoquinodimethane is synthesized through the condensation of 1,4-cyclohexanedione with malononitrile, followed by dehydrogenation of the resulting diene with bromine . The reaction can be summarized as follows:
Condensation Reaction:
Dehydrogenation Reaction:
化学反应分析
7,7,8,8-Tetracyanoquinodimethane undergoes various chemical reactions, including:
Charge Transfer Complex Formation:
相似化合物的比较
7,7,8,8-Tetracyanoquinodimethane is compared with other cyanocarbons and electron acceptors:
7,7,8,8-Tetracyanoquinodimethane stands out due to its ability to form highly conductive charge-transfer complexes, making it unique in the field of molecular electronics.
属性
IUPAC Name |
2-[4-(dicyanomethylidene)cyclohexa-2,5-dien-1-ylidene]propanedinitrile | |
---|---|---|
Source | PubChem | |
URL | https://pubchem.ncbi.nlm.nih.gov | |
Description | Data deposited in or computed by PubChem | |
InChI |
InChI=1S/C12H4N4/c13-5-11(6-14)9-1-2-10(4-3-9)12(7-15)8-16/h1-4H | |
Source | PubChem | |
URL | https://pubchem.ncbi.nlm.nih.gov | |
Description | Data deposited in or computed by PubChem | |
InChI Key |
PCCVSPMFGIFTHU-UHFFFAOYSA-N | |
Source | PubChem | |
URL | https://pubchem.ncbi.nlm.nih.gov | |
Description | Data deposited in or computed by PubChem | |
Canonical SMILES |
C1=CC(=C(C#N)C#N)C=CC1=C(C#N)C#N | |
Source | PubChem | |
URL | https://pubchem.ncbi.nlm.nih.gov | |
Description | Data deposited in or computed by PubChem | |
Molecular Formula |
C12H4N4 | |
Record name | tetracyanoquinodimethane | |
Source | Wikipedia | |
URL | https://en.wikipedia.org/wiki/Tetracyanoquinodimethane | |
Description | Chemical information link to Wikipedia. | |
Source | PubChem | |
URL | https://pubchem.ncbi.nlm.nih.gov | |
Description | Data deposited in or computed by PubChem | |
Related CAS |
26810-79-7 | |
Record name | Propanedinitrile, 2,2′-(2,5-cyclohexadiene-1,4-diylidene)bis-, homopolymer | |
Source | CAS Common Chemistry | |
URL | https://commonchemistry.cas.org/detail?cas_rn=26810-79-7 | |
Description | CAS Common Chemistry is an open community resource for accessing chemical information. Nearly 500,000 chemical substances from CAS REGISTRY cover areas of community interest, including common and frequently regulated chemicals, and those relevant to high school and undergraduate chemistry classes. This chemical information, curated by our expert scientists, is provided in alignment with our mission as a division of the American Chemical Society. | |
Explanation | The data from CAS Common Chemistry is provided under a CC-BY-NC 4.0 license, unless otherwise stated. | |
DSSTOX Substance ID |
DTXSID5061748 | |
Record name | Propanedinitrile, 2,2'-(2,5-cyclohexadiene-1,4-diylidene)bis- | |
Source | EPA DSSTox | |
URL | https://comptox.epa.gov/dashboard/DTXSID5061748 | |
Description | DSSTox provides a high quality public chemistry resource for supporting improved predictive toxicology. | |
Molecular Weight |
204.19 g/mol | |
Source | PubChem | |
URL | https://pubchem.ncbi.nlm.nih.gov | |
Description | Data deposited in or computed by PubChem | |
Physical Description |
Green crystalline solid; [Alfa Aesar MSDS] | |
Record name | Tetracyanoquinodimethane | |
Source | Haz-Map, Information on Hazardous Chemicals and Occupational Diseases | |
URL | https://haz-map.com/Agents/19839 | |
Description | Haz-Map® is an occupational health database designed for health and safety professionals and for consumers seeking information about the adverse effects of workplace exposures to chemical and biological agents. | |
Explanation | Copyright (c) 2022 Haz-Map(R). All rights reserved. Unless otherwise indicated, all materials from Haz-Map are copyrighted by Haz-Map(R). No part of these materials, either text or image may be used for any purpose other than for personal use. Therefore, reproduction, modification, storage in a retrieval system or retransmission, in any form or by any means, electronic, mechanical or otherwise, for reasons other than personal use, is strictly prohibited without prior written permission. | |
CAS No. |
1518-16-7 | |
Record name | Tetracyanoquinodimethane | |
Source | CAS Common Chemistry | |
URL | https://commonchemistry.cas.org/detail?cas_rn=1518-16-7 | |
Description | CAS Common Chemistry is an open community resource for accessing chemical information. Nearly 500,000 chemical substances from CAS REGISTRY cover areas of community interest, including common and frequently regulated chemicals, and those relevant to high school and undergraduate chemistry classes. This chemical information, curated by our expert scientists, is provided in alignment with our mission as a division of the American Chemical Society. | |
Explanation | The data from CAS Common Chemistry is provided under a CC-BY-NC 4.0 license, unless otherwise stated. | |
Record name | Tetracyanoquinodimethane | |
Source | ChemIDplus | |
URL | https://pubchem.ncbi.nlm.nih.gov/substance/?source=chemidplus&sourceid=0001518167 | |
Description | ChemIDplus is a free, web search system that provides access to the structure and nomenclature authority files used for the identification of chemical substances cited in National Library of Medicine (NLM) databases, including the TOXNET system. | |
Record name | TCNQ | |
Source | DTP/NCI | |
URL | https://dtp.cancer.gov/dtpstandard/servlet/dwindex?searchtype=NSC&outputformat=html&searchlist=105237 | |
Description | The NCI Development Therapeutics Program (DTP) provides services and resources to the academic and private-sector research communities worldwide to facilitate the discovery and development of new cancer therapeutic agents. | |
Explanation | Unless otherwise indicated, all text within NCI products is free of copyright and may be reused without our permission. Credit the National Cancer Institute as the source. | |
Record name | Propanedinitrile, 2,2'-(2,5-cyclohexadiene-1,4-diylidene)bis- | |
Source | EPA Chemicals under the TSCA | |
URL | https://www.epa.gov/chemicals-under-tsca | |
Description | EPA Chemicals under the Toxic Substances Control Act (TSCA) collection contains information on chemicals and their regulations under TSCA, including non-confidential content from the TSCA Chemical Substance Inventory and Chemical Data Reporting. | |
Record name | Propanedinitrile, 2,2'-(2,5-cyclohexadiene-1,4-diylidene)bis- | |
Source | EPA DSSTox | |
URL | https://comptox.epa.gov/dashboard/DTXSID5061748 | |
Description | DSSTox provides a high quality public chemistry resource for supporting improved predictive toxicology. | |
Record name | 2,2'-(2,5-cyclohexadiene-1,4-diylidene)bismalononitrile | |
Source | European Chemicals Agency (ECHA) | |
URL | https://echa.europa.eu/substance-information/-/substanceinfo/100.014.704 | |
Description | The European Chemicals Agency (ECHA) is an agency of the European Union which is the driving force among regulatory authorities in implementing the EU's groundbreaking chemicals legislation for the benefit of human health and the environment as well as for innovation and competitiveness. | |
Explanation | Use of the information, documents and data from the ECHA website is subject to the terms and conditions of this Legal Notice, and subject to other binding limitations provided for under applicable law, the information, documents and data made available on the ECHA website may be reproduced, distributed and/or used, totally or in part, for non-commercial purposes provided that ECHA is acknowledged as the source: "Source: European Chemicals Agency, http://echa.europa.eu/". Such acknowledgement must be included in each copy of the material. ECHA permits and encourages organisations and individuals to create links to the ECHA website under the following cumulative conditions: Links can only be made to webpages that provide a link to the Legal Notice page. | |
Record name | 7,7,8,8-TETRACYANO-P-QUINODIMETHANE | |
Source | FDA Global Substance Registration System (GSRS) | |
URL | https://gsrs.ncats.nih.gov/ginas/app/beta/substances/HC6FB4H2KW | |
Description | The FDA Global Substance Registration System (GSRS) enables the efficient and accurate exchange of information on what substances are in regulated products. Instead of relying on names, which vary across regulatory domains, countries, and regions, the GSRS knowledge base makes it possible for substances to be defined by standardized, scientific descriptions. | |
Explanation | Unless otherwise noted, the contents of the FDA website (www.fda.gov), both text and graphics, are not copyrighted. They are in the public domain and may be republished, reprinted and otherwise used freely by anyone without the need to obtain permission from FDA. Credit to the U.S. Food and Drug Administration as the source is appreciated but not required. | |
Synthesis routes and methods
Procedure details
Retrosynthesis Analysis
AI-Powered Synthesis Planning: Our tool employs the Template_relevance Pistachio, Template_relevance Bkms_metabolic, Template_relevance Pistachio_ringbreaker, Template_relevance Reaxys, Template_relevance Reaxys_biocatalysis model, leveraging a vast database of chemical reactions to predict feasible synthetic routes.
One-Step Synthesis Focus: Specifically designed for one-step synthesis, it provides concise and direct routes for your target compounds, streamlining the synthesis process.
Accurate Predictions: Utilizing the extensive PISTACHIO, BKMS_METABOLIC, PISTACHIO_RINGBREAKER, REAXYS, REAXYS_BIOCATALYSIS database, our tool offers high-accuracy predictions, reflecting the latest in chemical research and data.
Strategy Settings
Precursor scoring | Relevance Heuristic |
---|---|
Min. plausibility | 0.01 |
Model | Template_relevance |
Template Set | Pistachio/Bkms_metabolic/Pistachio_ringbreaker/Reaxys/Reaxys_biocatalysis |
Top-N result to add to graph | 6 |
Feasible Synthetic Routes
Q1: What is the molecular formula and weight of TCNQ?
A1: TCNQ's molecular formula is C12H4N4, and its molecular weight is 204.19 g/mol.
Q2: What are the key spectroscopic characteristics of TCNQ?
A2: TCNQ displays characteristic peaks in infrared (IR) spectroscopy, particularly the nitrile (C≡N) stretching frequency, which is sensitive to its oxidation state. [] This is particularly useful for analyzing charge transfer within TCNQ complexes. [, ] Additionally, Raman spectroscopy provides valuable information about vibrational modes, further aiding in characterizing TCNQ and its complexes. [, , ]
Q3: How does TCNQ interact with electron donors?
A3: TCNQ readily accepts electrons from donor molecules, forming charge-transfer complexes. [, , , ] The degree of charge transfer significantly influences the properties of these complexes, affecting their conductivity, magnetism, and optical characteristics. [, , , , , ]
Q4: What is the significance of TCNQ in material science?
A4: TCNQ is a versatile building block for creating diverse materials, including organic conductors, semiconductors, and magnetic materials. [, , , , , , , ] Its ability to form complexes with varying degrees of charge transfer makes it particularly attractive for tailoring material properties.
Q5: How does the morphology of TCNQ-based materials impact their properties?
A5: TCNQ-based materials exhibit a rich variety of morphologies, including nanowires, nanorods, and thin films. [, , , , ] The morphology significantly impacts properties like conductivity and switching behavior, making it a crucial factor in device fabrication. [, , ]
Q6: How does TCNQ interact with metal ions to form coordination polymers?
A6: TCNQ can coordinate to various metal ions, forming one-, two-, or three-dimensional coordination polymers. [, , , ] These polymers exhibit diverse structures depending on the metal ion, its oxidation state, and the presence of co-ligands. []
Q7: What are the catalytic applications of TCNQ and its derivatives?
A7: TCNQ and its derivatives, especially the fluorinated analogue TTF-TCNQF4, have shown promising catalytic activity in electron-transfer reactions, such as the reduction of ferricyanide ions by thiosulfate ions. [] The fluorinated derivative exhibits higher catalytic activity and remarkable stability, allowing for its recovery and reuse in multiple catalytic cycles. []
Q8: How is computational chemistry employed in understanding TCNQ-based systems?
A8: Density Functional Theory (DFT) calculations provide insights into the electronic structure, charge distribution, and intermolecular interactions within TCNQ-based materials. [, ] These calculations are crucial for interpreting experimental data and predicting the properties of novel TCNQ-based compounds.
Q9: How does fluorination of TCNQ (forming TCNQF4) affect its properties?
A9: Fluorination enhances the electron-accepting ability of TCNQ, leading to stronger charge-transfer interactions in complexes. [, , , ] This results in altered electronic properties, often leading to improved conductivity and catalytic activity compared to the parent TCNQ. [, ]
Q10: How does the structure of the donor molecule influence the properties of TCNQ-based charge transfer complexes?
A10: The size, shape, and electronic properties of the donor molecule significantly affect the packing arrangement and degree of charge transfer in TCNQ complexes. [, , ] These factors subsequently influence the conductivity, magnetism, and optical properties of the resulting materials. [, ]
Q11: What factors can influence the stability of TCNQ-based materials?
A11: Environmental factors like light and air exposure can impact the stability of TCNQ complexes. [] Additionally, the choice of solvent and the presence of water can affect the stability of doped complexes. [] Understanding these factors is critical for developing strategies to enhance material stability for various applications.
Q12: What analytical techniques are essential for characterizing TCNQ-based materials?
A12: A combination of techniques is employed to characterize TCNQ-based materials, including:
- X-ray diffraction (XRD): Provides information on crystal structure and phase identification. [, , , , ]
- Scanning Electron Microscopy (SEM): Reveals surface morphology and microstructure of the materials. [, , , , ]
- Atomic Force Microscopy (AFM): Offers high-resolution imaging of surface topography and nanostructure. []
- Electrochemical techniques: Cyclic voltammetry and chronoamperometry are used to study redox processes and kinetics. [, , ]
- Spectroscopic methods: UV-Vis, IR, and Raman spectroscopy provide information on electronic transitions, molecular vibrations, and charge transfer interactions. [, , , , , , , , ]
体外研究产品的免责声明和信息
请注意,BenchChem 上展示的所有文章和产品信息仅供信息参考。 BenchChem 上可购买的产品专为体外研究设计,这些研究在生物体外进行。体外研究,源自拉丁语 "in glass",涉及在受控实验室环境中使用细胞或组织进行的实验。重要的是要注意,这些产品没有被归类为药物或药品,他们没有得到 FDA 的批准,用于预防、治疗或治愈任何医疗状况、疾病或疾病。我们必须强调,将这些产品以任何形式引入人类或动物的身体都是法律严格禁止的。遵守这些指南对确保研究和实验的法律和道德标准的符合性至关重要。