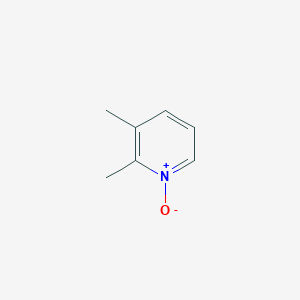
2,3-Dimethylpyridine 1-oxide
概述
描述
准备方法
Synthetic Routes and Reaction Conditions: The synthesis of 2,3-Dimethylpyridine 1-oxide typically involves the oxidation of 2,3-dimethylpyridine. One common method is the use of hydrogen peroxide as an oxidizing agent in the presence of acetic acid. The reaction is carried out under controlled conditions to ensure the selective formation of the N-oxide.
Industrial Production Methods: On an industrial scale, the production of this compound can be achieved through continuous flow processes, which allow for better control over reaction parameters and higher yields. The use of catalysts and optimized reaction conditions can further enhance the efficiency of the production process.
化学反应分析
Types of Reactions: 2,3-Dimethylpyridine 1-oxide undergoes various chemical reactions, including:
Oxidation: The compound can be further oxidized to form more complex N-oxide derivatives.
Reduction: Reduction reactions can convert the N-oxide back to the parent 2,3-dimethylpyridine.
Substitution: The methyl groups on the pyridine ring can undergo electrophilic substitution reactions.
Common Reagents and Conditions:
Oxidation: Hydrogen peroxide in acetic acid.
Reduction: Catalytic hydrogenation using palladium on carbon (Pd/C) as a catalyst.
Substitution: Electrophilic reagents such as halogens or nitro groups under acidic or basic conditions.
Major Products Formed:
Oxidation: Higher-order N-oxides.
Reduction: 2,3-Dimethylpyridine.
Substitution: Various substituted pyridine derivatives.
科学研究应用
2,3-Dimethylpyridine 1-oxide has several applications in scientific research:
Chemistry: It is used as a ligand in coordination chemistry and as a reagent in organic synthesis.
Biology: The compound is studied for its potential biological activities, including its role as an enzyme inhibitor.
Medicine: Research is ongoing to explore its potential as a therapeutic agent.
Industry: It is used in the synthesis of pharmaceuticals and agrochemicals.
作用机制
The mechanism of action of 2,3-Dimethylpyridine 1-oxide involves its interaction with molecular targets such as enzymes and receptors. The N-oxide group can participate in redox reactions, influencing the activity of enzymes and other proteins. The compound’s ability to form coordination complexes with metal ions also plays a role in its biological and chemical activities.
相似化合物的比较
- 2,4-Dimethylpyridine 1-oxide
- 2,5-Dimethylpyridine 1-oxide
- 2,6-Dimethylpyridine 1-oxide
- 3,4-Dimethylpyridine 1-oxide
- 3,5-Dimethylpyridine 1-oxide
Comparison: Compared to its isomers, 2,3-Dimethylpyridine 1-oxide exhibits unique reactivity due to the specific positioning of the methyl groups and the N-oxide. This positioning affects its electronic properties and steric hindrance, making it distinct in terms of its chemical behavior and applications.
属性
IUPAC Name |
2,3-dimethyl-1-oxidopyridin-1-ium | |
---|---|---|
Source | PubChem | |
URL | https://pubchem.ncbi.nlm.nih.gov | |
Description | Data deposited in or computed by PubChem | |
InChI |
InChI=1S/C7H9NO/c1-6-4-3-5-8(9)7(6)2/h3-5H,1-2H3 | |
Source | PubChem | |
URL | https://pubchem.ncbi.nlm.nih.gov | |
Description | Data deposited in or computed by PubChem | |
InChI Key |
QWLULCKKOHDCIE-UHFFFAOYSA-N | |
Source | PubChem | |
URL | https://pubchem.ncbi.nlm.nih.gov | |
Description | Data deposited in or computed by PubChem | |
Canonical SMILES |
CC1=C([N+](=CC=C1)[O-])C | |
Source | PubChem | |
URL | https://pubchem.ncbi.nlm.nih.gov | |
Description | Data deposited in or computed by PubChem | |
Molecular Formula |
C7H9NO | |
Source | PubChem | |
URL | https://pubchem.ncbi.nlm.nih.gov | |
Description | Data deposited in or computed by PubChem | |
Molecular Weight |
123.15 g/mol | |
Source | PubChem | |
URL | https://pubchem.ncbi.nlm.nih.gov | |
Description | Data deposited in or computed by PubChem | |
CAS No. |
22710-07-2 | |
Record name | Pyridine, 2,3-dimethyl-, 1-oxide | |
Source | CAS Common Chemistry | |
URL | https://commonchemistry.cas.org/detail?cas_rn=22710-07-2 | |
Description | CAS Common Chemistry is an open community resource for accessing chemical information. Nearly 500,000 chemical substances from CAS REGISTRY cover areas of community interest, including common and frequently regulated chemicals, and those relevant to high school and undergraduate chemistry classes. This chemical information, curated by our expert scientists, is provided in alignment with our mission as a division of the American Chemical Society. | |
Explanation | The data from CAS Common Chemistry is provided under a CC-BY-NC 4.0 license, unless otherwise stated. | |
Record name | Pyridine, 2,3-dimethyl-, 1-oxide | |
Source | European Chemicals Agency (ECHA) | |
URL | https://echa.europa.eu/information-on-chemicals | |
Description | The European Chemicals Agency (ECHA) is an agency of the European Union which is the driving force among regulatory authorities in implementing the EU's groundbreaking chemicals legislation for the benefit of human health and the environment as well as for innovation and competitiveness. | |
Explanation | Use of the information, documents and data from the ECHA website is subject to the terms and conditions of this Legal Notice, and subject to other binding limitations provided for under applicable law, the information, documents and data made available on the ECHA website may be reproduced, distributed and/or used, totally or in part, for non-commercial purposes provided that ECHA is acknowledged as the source: "Source: European Chemicals Agency, http://echa.europa.eu/". Such acknowledgement must be included in each copy of the material. ECHA permits and encourages organisations and individuals to create links to the ECHA website under the following cumulative conditions: Links can only be made to webpages that provide a link to the Legal Notice page. | |
Synthesis routes and methods I
Procedure details
Synthesis routes and methods II
Procedure details
Synthesis routes and methods III
Procedure details
Synthesis routes and methods IV
Procedure details
Synthesis routes and methods V
Procedure details
Retrosynthesis Analysis
AI-Powered Synthesis Planning: Our tool employs the Template_relevance Pistachio, Template_relevance Bkms_metabolic, Template_relevance Pistachio_ringbreaker, Template_relevance Reaxys, Template_relevance Reaxys_biocatalysis model, leveraging a vast database of chemical reactions to predict feasible synthetic routes.
One-Step Synthesis Focus: Specifically designed for one-step synthesis, it provides concise and direct routes for your target compounds, streamlining the synthesis process.
Accurate Predictions: Utilizing the extensive PISTACHIO, BKMS_METABOLIC, PISTACHIO_RINGBREAKER, REAXYS, REAXYS_BIOCATALYSIS database, our tool offers high-accuracy predictions, reflecting the latest in chemical research and data.
Strategy Settings
Precursor scoring | Relevance Heuristic |
---|---|
Min. plausibility | 0.01 |
Model | Template_relevance |
Template Set | Pistachio/Bkms_metabolic/Pistachio_ringbreaker/Reaxys/Reaxys_biocatalysis |
Top-N result to add to graph | 6 |
Feasible Synthetic Routes
体外研究产品的免责声明和信息
请注意,BenchChem 上展示的所有文章和产品信息仅供信息参考。 BenchChem 上可购买的产品专为体外研究设计,这些研究在生物体外进行。体外研究,源自拉丁语 "in glass",涉及在受控实验室环境中使用细胞或组织进行的实验。重要的是要注意,这些产品没有被归类为药物或药品,他们没有得到 FDA 的批准,用于预防、治疗或治愈任何医疗状况、疾病或疾病。我们必须强调,将这些产品以任何形式引入人类或动物的身体都是法律严格禁止的。遵守这些指南对确保研究和实验的法律和道德标准的符合性至关重要。