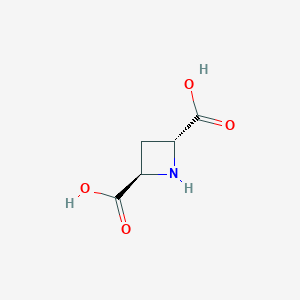
氮杂环丁-2,4-二羧酸
描述
Synthesis Analysis
The synthesis of azetidine derivatives, including azetidine-2,4-dicarboxylic acid, has been explored through several efficient methods. One notable approach involves the palladium-catalyzed intramolecular amination of C(sp3)-H and C(sp2)-H bonds at γ and δ positions, which highlights the use of unactivated C-H bonds, especially the C(sp(3))-H bond of methyl groups, as functional groups in organic synthesis (He et al., 2012). Another method reported is a novel, simple, clean, and improved procedure starting from γ-butyrolactone, achieving an overall yield of 13.2% (Jin & Zhang, 2012).
Molecular Structure Analysis
Azetidines, including azetidine-2,4-dicarboxylic acid, are characterized by their strained four-membered ring structure, which significantly influences their reactivity. The presence of the nitrogen atom in the ring contributes to the compound's basicity and nucleophilicity. This structural attribute facilitates its involvement in various chemical transformations, making it a valuable building block in organic synthesis.
Chemical Reactions and Properties
Azetidine derivatives are known for their involvement in a range of chemical reactions, leveraging their ring strain for synthetic applications. For instance, functionalized azetidine derivatives have been synthesized for potential interest in biological and foldameric applications, showcasing the versatility of these compounds in chemical synthesis (Žukauskaitė et al., 2011). Moreover, the synthesis of enantiopure azetidine derivatives through stereoselective methodologies has been demonstrated, highlighting the compound's utility in constructing chirally pure structures (Drouillat et al., 2012).
科学研究应用
NMDA 受体调节:氮杂环丁-2,4-二羧酸衍生物已被合成,并被发现能增强谷氨酸、天冬氨酸或 N-甲基-D-天冬氨酸 (NMDA) 在小脑颗粒细胞中刺激 NMDA 受体的钙摄取。这表明其在调节 NMDA 受体活性中的潜在作用,这对于研究神经过程和疾病具有重要意义 (Kozikowski 等,1990)。
植物中的离子转运:氮杂环丁-2-羧酸已被用作脯氨酸的类似物来研究植物中的蛋白质合成与离子转运之间的关系。发现它是大麦根中离子释放到木质部的一种有效抑制剂,从而深入了解植物中离子转运的机制 (Pitman 等,1977)。
代谢型谷氨酸受体:氮杂环丁-2,4-二羧酸已针对其在人代谢型谷氨酸受体中的活性进行了分析。它在人 mGlu2 受体中表现出微弱的激动剂活性,但在人 mGlu4a、mGlu1b 和 mGlu5a 受体中没有活性。这为理解其与谷氨酸受体相关的药理学特征提供了基础 (Knöpfel 等,1995)。
蛋白质合成和构象:氮杂环丁-2-羧酸已用于脯氨酸代谢和蛋白质构象的研究中。这包括它在蛋白质中的掺入及其对蛋白质折叠的影响,这对于理解各种细胞过程至关重要 (Verbruggen 等,1992)。
毒性和致畸性:氮杂环丁-2-羧酸 (L-Aze) 已被确定为一种有毒且致畸的非蛋白质氨基酸。它可以取代脯氨酸错误掺入蛋白质中,从而导致胶原、角蛋白、血红蛋白和蛋白质折叠发生改变。这在致畸作用和人类疾病中具有影响 (Rubenstein 等,2006)。
癌症治疗中的放射增敏剂:氮杂环丁-2-羧酸已被证明是肝癌细胞治疗中超热和电离辐射的有效增敏剂。这表明其在提高癌症治疗效果中的潜在用途 (van Rijn 等,1999)。
合成化学和应用:氮杂环丁,包括氮杂环丁-2-羧酸,在合成化学中很重要,在肽模拟物和核酸化学以及催化过程中有应用 (Mehra 等,2017)。
作用机制
未来方向
Azetidines represent one of the most important four-membered heterocycles used in organic synthesis and medicinal chemistry . Recent advances in the chemistry and reactivity of azetidines have been reported, with a focus on the most recent advances, trends, and future directions . Azetidine-2,4-dicarboxylic acid might prove useful in differentiating the function of various mGluR subtypes, which could have implications for drug discovery .
属性
IUPAC Name |
(2R,4R)-azetidine-2,4-dicarboxylic acid | |
---|---|---|
Source | PubChem | |
URL | https://pubchem.ncbi.nlm.nih.gov | |
Description | Data deposited in or computed by PubChem | |
InChI |
InChI=1S/C5H7NO4/c7-4(8)2-1-3(6-2)5(9)10/h2-3,6H,1H2,(H,7,8)(H,9,10)/t2-,3-/m1/s1 | |
Source | PubChem | |
URL | https://pubchem.ncbi.nlm.nih.gov | |
Description | Data deposited in or computed by PubChem | |
InChI Key |
JMVIGOFRIJJUAW-PWNYCUMCSA-N | |
Source | PubChem | |
URL | https://pubchem.ncbi.nlm.nih.gov | |
Description | Data deposited in or computed by PubChem | |
Canonical SMILES |
C1C(NC1C(=O)O)C(=O)O | |
Source | PubChem | |
URL | https://pubchem.ncbi.nlm.nih.gov | |
Description | Data deposited in or computed by PubChem | |
Isomeric SMILES |
C1[C@@H](N[C@H]1C(=O)O)C(=O)O | |
Source | PubChem | |
URL | https://pubchem.ncbi.nlm.nih.gov | |
Description | Data deposited in or computed by PubChem | |
Molecular Formula |
C5H7NO4 | |
Source | PubChem | |
URL | https://pubchem.ncbi.nlm.nih.gov | |
Description | Data deposited in or computed by PubChem | |
DSSTOX Substance ID |
DTXSID60925846 | |
Record name | Azetidine-2,4-dicarboxylic acid | |
Source | EPA DSSTox | |
URL | https://comptox.epa.gov/dashboard/DTXSID60925846 | |
Description | DSSTox provides a high quality public chemistry resource for supporting improved predictive toxicology. | |
Molecular Weight |
145.11 g/mol | |
Source | PubChem | |
URL | https://pubchem.ncbi.nlm.nih.gov | |
Description | Data deposited in or computed by PubChem | |
Product Name |
Azetidine-2,4-dicarboxylic acid | |
CAS RN |
127310-57-0 | |
Record name | Azetidine-2,4-dicarboxylic acid | |
Source | ChemIDplus | |
URL | https://pubchem.ncbi.nlm.nih.gov/substance/?source=chemidplus&sourceid=0127310570 | |
Description | ChemIDplus is a free, web search system that provides access to the structure and nomenclature authority files used for the identification of chemical substances cited in National Library of Medicine (NLM) databases, including the TOXNET system. | |
Record name | Azetidine-2,4-dicarboxylic acid | |
Source | EPA DSSTox | |
URL | https://comptox.epa.gov/dashboard/DTXSID60925846 | |
Description | DSSTox provides a high quality public chemistry resource for supporting improved predictive toxicology. | |
Retrosynthesis Analysis
AI-Powered Synthesis Planning: Our tool employs the Template_relevance Pistachio, Template_relevance Bkms_metabolic, Template_relevance Pistachio_ringbreaker, Template_relevance Reaxys, Template_relevance Reaxys_biocatalysis model, leveraging a vast database of chemical reactions to predict feasible synthetic routes.
One-Step Synthesis Focus: Specifically designed for one-step synthesis, it provides concise and direct routes for your target compounds, streamlining the synthesis process.
Accurate Predictions: Utilizing the extensive PISTACHIO, BKMS_METABOLIC, PISTACHIO_RINGBREAKER, REAXYS, REAXYS_BIOCATALYSIS database, our tool offers high-accuracy predictions, reflecting the latest in chemical research and data.
Strategy Settings
Precursor scoring | Relevance Heuristic |
---|---|
Min. plausibility | 0.01 |
Model | Template_relevance |
Template Set | Pistachio/Bkms_metabolic/Pistachio_ringbreaker/Reaxys/Reaxys_biocatalysis |
Top-N result to add to graph | 6 |
Feasible Synthetic Routes
Q & A
Q1: How does Azetidine-2,4-dicarboxylic acid interact with its target and what are the downstream effects?
A: Azetidine-2,4-dicarboxylic acid primarily interacts with metabotropic glutamate receptors (mGluRs) [, , , , , , , , , ]. It acts as an agonist at specific mGluR subtypes, stimulating phosphoinositide hydrolysis, a key signaling pathway in neurons [, ]. This activation can lead to various downstream effects, including modulation of long-term potentiation (LTP), a cellular mechanism for learning and memory [, ], and inhibition of glutamate release from microglia, potentially protecting neurons from excitotoxicity [].
Q2: What is known about the structure of Azetidine-2,4-dicarboxylic acid?
A: Azetidine-2,4-dicarboxylic acid is a rigid analog of glutamate [, ]. It exists as cis and trans isomers, with the cis isomer showing higher potency at NMDA receptors []. The synthesis of optically active forms has been achieved using chiral auxiliaries like (S)-1-phenylethylamine, allowing for the determination of absolute configuration through X-ray crystallography [].
Q3: How does Azetidine-2,4-dicarboxylic acid influence long-term potentiation (LTP)?
A: Studies show that Azetidine-2,4-dicarboxylic acid can both facilitate and inhibit LTP depending on the experimental conditions. For instance, intracerebroventricular injection of Azetidine-2,4-dicarboxylic acid prolonged LTP induced by weak tetanization in the dentate gyrus of rats [, ]. This effect was blocked by the mGluR antagonist (R,S)-α-methyl-4-carboxyphenylglycine, suggesting it was mediated by mGluR activation [].
Q4: What is the role of Azetidine-2,4-dicarboxylic acid in excitotoxicity?
A: Azetidine-2,4-dicarboxylic acid has shown neuroprotective effects against glutamate and NMDA-induced excitotoxicity in primary cultures of cerebellar neurons []. This protection is attributed to the activation of mGluR5, which counteracts the excitotoxic cascade []. Additionally, by inhibiting glutamate release from activated microglia, Azetidine-2,4-dicarboxylic acid indirectly prevents excessive glutamate levels that contribute to neuronal death [].
Q5: Are there any Structure-Activity Relationship (SAR) studies on Azetidine-2,4-dicarboxylic acid?
A: While detailed SAR studies on Azetidine-2,4-dicarboxylic acid itself are limited in the provided research, related studies exploring derivatives of this compound offer valuable insights [, ]. Modifications such as esterification and N-methylation have been investigated for their impact on activity and selectivity towards different glutamate receptor subtypes [, ].
Q6: What in vivo studies have been conducted on Azetidine-2,4-dicarboxylic acid?
A: Azetidine-2,4-dicarboxylic acid has been studied in vivo in rats. Intracerebroventricular injections were used to investigate its effects on LTP in the dentate gyrus [, ] and its behavioral effects in the open field test []. These studies highlight the potential of Azetidine-2,4-dicarboxylic acid to modulate neuronal activity and behavior.
体外研究产品的免责声明和信息
请注意,BenchChem 上展示的所有文章和产品信息仅供信息参考。 BenchChem 上可购买的产品专为体外研究设计,这些研究在生物体外进行。体外研究,源自拉丁语 "in glass",涉及在受控实验室环境中使用细胞或组织进行的实验。重要的是要注意,这些产品没有被归类为药物或药品,他们没有得到 FDA 的批准,用于预防、治疗或治愈任何医疗状况、疾病或疾病。我们必须强调,将这些产品以任何形式引入人类或动物的身体都是法律严格禁止的。遵守这些指南对确保研究和实验的法律和道德标准的符合性至关重要。