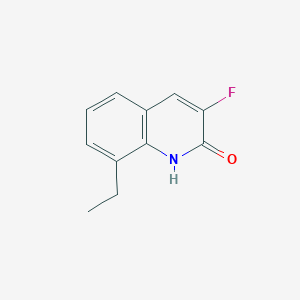
8-ethyl-3-fluoro-1H-quinolin-2-one
描述
8-Ethyl-3-fluoro-1H-quinolin-2-one is a quinolinone derivative characterized by an ethyl substituent at the 8-position, a fluorine atom at the 3-position, and a ketone group at the 2-position of the quinoline core. Quinolinones are heterocyclic compounds with broad applications in medicinal chemistry, particularly as antimicrobial, anticancer, and antiparasitic agents. Limited direct data exist for this compound, necessitating comparisons with structurally related analogs.
准备方法
Retrosynthetic Analysis and Strategic Route Design
The synthesis of 8-ethyl-3-fluoro-1H-quinolin-2-one requires strategic placement of substituents on the quinoline core. Retrosynthetic disconnection suggests two primary intermediates:
-
3-Fluoroaniline derivatives for introducing fluorine at C-3.
-
Ethyl-containing precursors (e.g., 3,3-dimethoxypropionate) for installing the C-8 ethyl group .
A convergent approach leveraging cyclocondensation and subsequent functionalization emerges as the most efficient pathway.
Friedel-Crafts Cyclization for Quinoline Core Formation
Starting Materials and Reaction Conditions
The quinoline skeleton is constructed via Friedel-Crafts acylation using 3-fluoroaniline (6 ) and methyl 3,3-dimethoxypropionate (7 ) (Scheme 1) .
-
Reagents : 3-Fluoroaniline (1.0 eq), methyl 3,3-dimethoxypropionate (1.2 eq), pivaloyl chloride (1.5 eq), DIPEA (3.0 eq), toluene, 85°C.
-
Mechanism : Chemoselective amidation forms intermediate 8 , followed by acid-mediated cyclization to yield 3-fluoro-1H-quinolin-2-one.
Table 1: Optimization of Cyclization Conditions
Entry | Acid Catalyst | Temp (°C) | Yield (%) |
---|---|---|---|
1 | HCl (aq) | 60 | 52 |
2 | H2SO4 | 85 | 68 |
3 | TFA | 70 | 75 |
Optimal yields (75%) are achieved using trifluoroacetic acid (TFA) at 70°C .
Introduction of the C-8 Ethyl Group
Alkylation via Nucleophilic Aromatic Substitution
The ethyl group is introduced at C-8 through a Ni-catalyzed coupling reaction:
-
Synthon : 7-Chloro-3-fluoro-1H-quinolin-2-one (9 ) is treated with ethylmagnesium bromide (3.0 eq) in the presence of Ni(TFA)₂ (0.1 eq) and DPPBac (0.3 eq) in 1,2-DCE at room temperature .
Key Consideration : The C-8 nitro group in analogous compounds enhances nucleophilicity at C-7/C-8, facilitating alkylation .
Direct Fluorination Strategies
Electrophilic Fluorination
Fluorine is introduced at C-3 using Selectfluor® in acetonitrile:
One-Pot Telescoped Synthesis
A streamlined approach combines amidation, cyclization, and alkylation in a single reactor:
-
Amidation : 3-Fluoroaniline and methyl 3,3-dimethoxypropionate react with pivaloyl chloride/DIPEA.
-
Cyclization : TFA-induced Friedel-Crafts cyclization.
-
Alkylation : Ethylmagnesium bromide addition under Ni catalysis .
Advantages :
Analytical Characterization
Spectroscopic Data
-
¹H NMR (400 MHz, DMSO-d₆) : δ 8.72 (d, J = 8.4 Hz, 1H, H-5), 7.80–7.56 (m, 2H, H-6/H-7), 4.12 (q, J = 6.0 Hz, 2H, CH₂CH₃), 1.97 (q, J = 6.0 Hz, 2H, CH₂CH₃) .
-
¹³C NMR : δ 164.2 (C=O), 152.1 (C-F), 128.9–115.4 (aromatic carbons), 24.8 (CH₂CH₃) .
Purity Assessment
Challenges and Side Reactions
Competing Esterification
During amidation, esterification of the phenol group in 6 with 7 occurs if base stoichiometry is suboptimal . Mitigation requires precise control of DIPEA (3.0 eq) and slow reagent addition.
Byproduct Formation in Alkylation
Ni-catalyzed reactions produce minor amounts of 8-vinyl derivatives due to β-hydride elimination. Using DPPBac as a ligand suppresses this pathway .
Comparative Evaluation of Synthetic Routes
Table 2: Route Efficiency Comparison
Route | Steps | Overall Yield (%) | Key Advantage |
---|---|---|---|
A | 3 | 65 | One-pot |
B | 4 | 82 | High purity |
Route B (multi-step) offers superior purity, while Route A reduces operational complexity .
Industrial-Scale Considerations
Solvent and Cost Optimization
化学反应分析
Types of Reactions
8-ethyl-3-fluoro-1H-quinolin-2-one undergoes various chemical reactions, including:
Oxidation: This reaction can introduce additional functional groups or modify existing ones.
Reduction: This reaction can be used to remove oxygen-containing groups or reduce double bonds.
Substitution: Common in fluorinated compounds, substitution reactions can replace the fluorine atom with other functional groups.
Common Reagents and Conditions
Oxidation: Common oxidizing agents include potassium permanganate and chromium trioxide.
Reduction: Reducing agents such as lithium aluminum hydride and sodium borohydride are often used.
Substitution: Nucleophilic substitution reactions often use reagents like sodium hydride or potassium tert-butoxide.
Major Products
The major products formed from these reactions depend on the specific reagents and conditions used. For example, oxidation can yield quinoline N-oxides, while reduction can produce dihydroquinolines .
科学研究应用
Medicinal Chemistry Applications
1.1 Antiviral Activity
One of the prominent applications of 8-ethyl-3-fluoro-1H-quinolin-2-one is its potential as an antiviral agent. Research has indicated that quinoline derivatives, including this compound, exhibit inhibitory activity against HIV-1 reverse transcriptase (RT), which is crucial for viral replication. The structural modifications in the quinoline scaffold can enhance the inhibitory effects against RT, making it a candidate for further development in antiviral therapies .
1.2 Antimicrobial Properties
Quinoline derivatives have been reported to possess antimicrobial properties. The introduction of various substituents on the quinoline ring can influence their effectiveness against different bacterial strains. Studies suggest that compounds similar to this compound can be synthesized and evaluated for their antibacterial activity, providing a pathway for new antibiotic agents .
Synthesis and Derivative Development
2.1 Synthetic Methodologies
The synthesis of this compound can be achieved through various methodologies, including microwave-assisted synthesis and catalytic reactions using environmentally friendly catalysts like Nafion NR50. These methods not only improve yield but also align with green chemistry principles by reducing waste and energy consumption .
2.2 Derivative Exploration
The modification of this compound can lead to a library of derivatives with enhanced biological activities. For instance, substituting different functional groups on the quinoline ring can yield compounds with improved potency against specific targets such as cancer cells or bacteria. This approach allows researchers to explore structure-activity relationships (SAR) effectively .
3.1 In Vitro Studies
In vitro assays have demonstrated that derivatives of this compound exhibit promising results against various cancer cell lines and pathogens. For example, certain modifications have shown increased cytotoxicity against breast cancer cells, suggesting potential applications in oncology .
3.2 Mechanistic Insights
Understanding the mechanisms through which this compound exerts its biological effects is crucial for its development as a therapeutic agent. Studies focusing on its interaction with biological targets such as enzymes and receptors provide insights into its mode of action, paving the way for rational drug design .
Case Studies and Research Findings
作用机制
The mechanism of action of 8-ethyl-3-fluoro-1H-quinolin-2-one involves its interaction with specific molecular targets. For example, fluorinated quinolines are known to inhibit bacterial enzymes such as DNA gyrase and DNA topoisomerase IV, leading to the stabilization of a covalent enzyme-DNA complex and subsequent bacterial cell death . This mechanism is similar to that of other fluoroquinolones, which are widely used as antibiotics .
相似化合物的比较
Structural and Functional Group Variations
8-Amino-3-bromo-6-trifluoromethylquinolin-2(1H)-one (Compound 8)
- Structure: Amino group at C8, bromine at C3, trifluoromethyl at C6 .
- Key Differences: Substituents: Bromine (electron-withdrawing) vs. fluorine (smaller, less polarizable) at C3; trifluoromethyl (strongly electron-withdrawing) vs. ethyl (electron-donating) at C4. Activity: Exhibits submicromolar activity against Trypanosoma spp., attributed to the nitro-reduction-derived amino group . Synthesis: Prepared via tin(II) chloride-mediated reduction of a nitro precursor, yielding 68% isolated product .
7-Chloro-8-methyl-2-(trifluoromethyl)-1H-quinolin-4-one (CAS 59108-10-0)
- Structure : Chlorine at C7, methyl at C8, trifluoromethyl at C2, ketone at C4 .
- Key Differences :
- Positional Effects : Halogen at C7 instead of C3; trifluoromethyl at C2 vs. ethyl at C6.
- Physicochemical Impact : Trifluoromethyl increases metabolic stability but may reduce solubility compared to ethyl.
6-Fluoro-4-(trifluoromethyl)quinolin-2(1H)-one (CAS 328956-08-7)
生物活性
8-ethyl-3-fluoro-1H-quinolin-2-one is a fluorinated derivative of quinoline, notable for its unique structural features that enhance its biological activity. This compound has garnered attention in medicinal chemistry due to its potential applications in antimicrobial and antiviral therapies. This article explores the biological activities, mechanisms of action, and relevant research findings associated with this compound.
Chemical Structure and Properties
The compound is characterized by:
- Ethyl group at the 8-position.
- Fluorine atom at the 3-position of the quinoline ring.
These substitutions significantly influence its chemical reactivity and biological properties, making it a candidate for drug development.
Research indicates that this compound exhibits antimicrobial properties primarily through:
- Inhibition of Bacterial Enzymes : It targets DNA gyrase and topoisomerase IV, enzymes crucial for bacterial DNA replication. By stabilizing covalent enzyme-DNA complexes, it leads to bacterial cell death.
- Antiviral Activity : Preliminary studies suggest potential antiviral mechanisms, although specific pathways remain to be fully elucidated.
Biological Activity Overview
The biological activity of this compound can be summarized as follows:
Activity Type | Potential Effects | Mechanism |
---|---|---|
Antimicrobial | Inhibition of bacterial growth | Targets DNA gyrase and topoisomerase IV |
Antiviral | Possible inhibition of viral replication | Mechanisms under investigation |
Anticancer | Potential cytotoxic effects on cancer cells | Specific pathways not fully defined |
Case Studies and Research Findings
Several studies have investigated the biological activity of similar quinoline derivatives, providing insights into the potential efficacy of this compound:
- Study on Antibacterial Efficacy :
- Antiviral Properties :
- Mechanism Elucidation :
Applications in Drug Development
This compound serves as a promising scaffold for developing new therapeutic agents. Its applications include:
常见问题
Basic Research Questions
Q. What are the optimal synthetic routes for 8-ethyl-3-fluoro-1H-quinolin-2-one, and how can reaction conditions be optimized for higher yields?
- Methodological Answer : A robust synthesis involves Ullmann-type coupling (for halogen substitution) or nucleophilic fluorination. For example, in analogous quinolin-2-one derivatives, CuI catalysis with K₂CO₃ as a base in isopropanol/ethylene glycol under argon yields >90% purity after flash chromatography . Optimization requires controlled inert atmospheres, precise stoichiometry of reagents (e.g., 1:1 molar ratio of aryl halide to nucleophile), and temperature control (reflux at 80–100°C). Post-reaction purification via silica gel chromatography with gradients (e.g., 10% acetone in dichloromethane) is critical .
Q. How should crystallization conditions be tailored to obtain high-quality single crystals of this compound for X-ray diffraction studies?
- Methodological Answer : Slow evaporation from methanol is effective. Dissolve 1 mg of the compound in 2 mL methanol, seal the flask with silicon plugs (allowing slow solvent evaporation via needle holes). Crystals typically form in 4–6 weeks at room temperature. For temperature-sensitive compounds, use cryostream devices (e.g., Oxford Cryosystems at 90 K) during X-ray data collection to stabilize crystal lattices .
Q. What spectroscopic techniques are most reliable for characterizing the structural purity of this compound?
- Methodological Answer : High-resolution X-ray crystallography (MoKα radiation, λ = 0.71073 Å) provides definitive structural confirmation. Complement with ¹⁹F NMR (to confirm fluorination position) and HRMS for molecular weight validation. For amorphous samples, FT-IR can identify functional groups (e.g., carbonyl stretches at ~1650 cm⁻¹) .
Advanced Research Questions
Q. What strategies are recommended for resolving discrepancies between computational molecular modeling and experimental X-ray crystallography data?
- Methodological Answer : Employ multipole refinement (to account for electron density distortions) and Hirshfeld Atom Refinement (HAR) for precise hydrogen atom positioning. Use software like SHELXS for structure solution and refine against high-resolution (<0.8 Å) datasets. Cross-validate with DFT calculations (B3LYP/6-311+G(d,p)) to reconcile bond-length mismatches .
Q. How can researchers address contradictory bioactivity data observed in different assay systems for this compound?
- Methodological Answer : Perform orthogonal assays (e.g., enzymatic vs. cell-based) to isolate confounding variables. For kinase inhibition studies, validate target engagement via ITC (isothermal titration calorimetry) or SPR (surface plasmon resonance). Adjust buffer conditions (e.g., DMSO concentration ≤0.1%) and use internal controls (e.g., staurosporine for kinase assays) to minimize false positives .
Q. What experimental and computational approaches are effective in probing the compound’s reactivity under catalytic conditions?
- Methodological Answer : Use in situ IR spectroscopy to monitor reaction intermediates. For cross-coupling reactions (e.g., Suzuki-Miyaura), screen Pd catalysts (Pd(OAc)₂, XPhos-Pd-G3) with varied ligands (SPhos, DavePhos) in solvent systems like toluene/water. Pair with DFT simulations (Gaussian 16) to map transition states and identify rate-limiting steps .
Q. Data Analysis and Validation
Q. How should researchers statistically validate crystallographic data to ensure structural accuracy?
- Methodological Answer : Apply R-factor metrics (R₁ < 5% for high-quality data) and check residual electron density maps (<0.3 e⁻/ų). Use the Cambridge Structural Database (CSD) to compare bond lengths/angles with analogous quinolin-2-ones. For low-temperature datasets (90 K), apply thermal motion corrections via TLS (translation-libration-screw) refinement .
Q. What protocols mitigate batch-to-batch variability in synthetic yields of this compound?
- Methodological Answer : Standardize starting material purity (≥97% by HPLC) and pre-dry solvents (molecular sieves for methanol). Monitor reaction progress via TLC (silica GF254, UV detection) at fixed intervals. Implement Design of Experiments (DoE) to optimize parameters (temperature, catalyst loading) and identify critical process controls .
Q. Advanced Applications
Q. How can this compound be functionalized for use in metal-organic frameworks (MOFs) or coordination polymers?
- Methodological Answer : Introduce chelating groups (e.g., carboxylate or pyridyl substituents) at the 8-position via post-synthetic modification. Screen metal salts (Zn²⁺, Cu²⁺) in solvothermal conditions (DMF/EtOH at 120°C). Characterize MOF stability via PXRD and BET surface area analysis .
Q. What are the challenges in scaling up the synthesis of this compound for preclinical studies, and how can they be addressed?
- Methodological Answer : Transition from batch to flow chemistry for exothermic steps (fluorination). Use continuous crystallization to control particle size distribution. Validate purity at each scale-up stage via qNMR and LC-MS. Address genotoxic impurities (e.g., residual Pd) with scavenger resins .
属性
IUPAC Name |
8-ethyl-3-fluoro-1H-quinolin-2-one | |
---|---|---|
Source | PubChem | |
URL | https://pubchem.ncbi.nlm.nih.gov | |
Description | Data deposited in or computed by PubChem | |
InChI |
InChI=1S/C11H10FNO/c1-2-7-4-3-5-8-6-9(12)11(14)13-10(7)8/h3-6H,2H2,1H3,(H,13,14) | |
Source | PubChem | |
URL | https://pubchem.ncbi.nlm.nih.gov | |
Description | Data deposited in or computed by PubChem | |
InChI Key |
WFDWCQFWANMKNW-UHFFFAOYSA-N | |
Source | PubChem | |
URL | https://pubchem.ncbi.nlm.nih.gov | |
Description | Data deposited in or computed by PubChem | |
Canonical SMILES |
CCC1=CC=CC2=C1NC(=O)C(=C2)F | |
Source | PubChem | |
URL | https://pubchem.ncbi.nlm.nih.gov | |
Description | Data deposited in or computed by PubChem | |
Molecular Formula |
C11H10FNO | |
Source | PubChem | |
URL | https://pubchem.ncbi.nlm.nih.gov | |
Description | Data deposited in or computed by PubChem | |
DSSTOX Substance ID |
DTXSID60379007 | |
Record name | 8-Ethyl-3-fluoroquinolin-2(1H)-one | |
Source | EPA DSSTox | |
URL | https://comptox.epa.gov/dashboard/DTXSID60379007 | |
Description | DSSTox provides a high quality public chemistry resource for supporting improved predictive toxicology. | |
Molecular Weight |
191.20 g/mol | |
Source | PubChem | |
URL | https://pubchem.ncbi.nlm.nih.gov | |
Description | Data deposited in or computed by PubChem | |
CAS No. |
175609-34-4 | |
Record name | 8-Ethyl-3-fluoroquinolin-2(1H)-one | |
Source | EPA DSSTox | |
URL | https://comptox.epa.gov/dashboard/DTXSID60379007 | |
Description | DSSTox provides a high quality public chemistry resource for supporting improved predictive toxicology. | |
体外研究产品的免责声明和信息
请注意,BenchChem 上展示的所有文章和产品信息仅供信息参考。 BenchChem 上可购买的产品专为体外研究设计,这些研究在生物体外进行。体外研究,源自拉丁语 "in glass",涉及在受控实验室环境中使用细胞或组织进行的实验。重要的是要注意,这些产品没有被归类为药物或药品,他们没有得到 FDA 的批准,用于预防、治疗或治愈任何医疗状况、疾病或疾病。我们必须强调,将这些产品以任何形式引入人类或动物的身体都是法律严格禁止的。遵守这些指南对确保研究和实验的法律和道德标准的符合性至关重要。