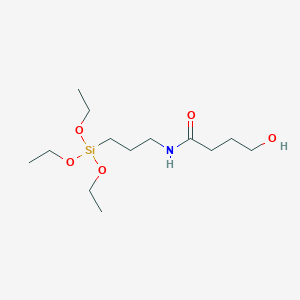
N-(3-Triethoxysilylpropyl)-4-hydroxybutyramide
描述
N-(3-Triethoxysilylpropyl)-4-hydroxybutyramide is an organosilane compound that features both silane and amide functional groups. This compound is known for its ability to form strong bonds with both organic and inorganic materials, making it a valuable component in various industrial and scientific applications. The presence of the triethoxysilyl group allows it to participate in silanization reactions, which are crucial for surface modification and adhesion promotion.
准备方法
Synthetic Routes and Reaction Conditions
The synthesis of N-(3-Triethoxysilylpropyl)-4-hydroxybutyramide typically involves the reaction of 3-aminopropyltriethoxysilane with 4-hydroxybutyric acid. The reaction is carried out under anhydrous conditions to prevent hydrolysis of the triethoxysilyl group. The process generally involves the following steps:
Reaction Setup: The reactants are dissolved in an appropriate solvent, such as toluene or tetrahydrofuran.
Reaction Conditions: The mixture is heated to a temperature range of 60-80°C and stirred for several hours.
Purification: The product is purified using techniques such as distillation or chromatography to remove any unreacted starting materials and by-products.
Industrial Production Methods
In an industrial setting, the production of this compound may involve continuous flow reactors to ensure consistent quality and yield. The use of automated systems allows for precise control over reaction conditions, such as temperature, pressure, and reactant concentrations. Additionally, large-scale purification methods, such as crystallization and solvent extraction, are employed to obtain high-purity products.
化学反应分析
Types of Reactions
N-(3-Triethoxysilylpropyl)-4-hydroxybutyramide undergoes various chemical reactions, including:
Hydrolysis: The triethoxysilyl group can be hydrolyzed in the presence of water, leading to the formation of silanol groups.
Condensation: The silanol groups formed from hydrolysis can further condense to form siloxane bonds, which are essential for cross-linking and network formation.
Common Reagents and Conditions
Hydrolysis: Typically carried out in the presence of a catalyst, such as hydrochloric acid or acetic acid, at room temperature.
Condensation: Often facilitated by heating the reaction mixture to 100-150°C in the presence of a catalyst, such as titanium isopropoxide.
Substitution: Requires the use of nucleophiles, such as amines or alcohols, under mild heating conditions.
Major Products
Siloxane Networks: Formed through the condensation of silanol groups.
Functionalized Amides: Resulting from nucleophilic substitution reactions at the amide group.
科学研究应用
N-(3-Triethoxysilylpropyl)-4-hydroxybutyramide has a wide range of applications in scientific research, including:
Surface Modification: Used to functionalize surfaces of materials, such as glass, metals, and polymers, to enhance adhesion and compatibility.
Bioconjugation: Employed in the attachment of biomolecules to surfaces for biosensor and diagnostic applications.
Nanotechnology: Utilized in the synthesis of hybrid nanomaterials with improved mechanical and thermal properties.
Catalysis: Acts as a ligand in the preparation of catalysts for various organic transformations.
Drug Delivery: Explored for its potential in the development of targeted drug delivery systems due to its ability to form stable bonds with biological molecules.
作用机制
The mechanism of action of N-(3-Triethoxysilylpropyl)-4-hydroxybutyramide involves the formation of strong covalent bonds with both organic and inorganic substrates. The triethoxysilyl group undergoes hydrolysis to form silanol groups, which can then condense to form siloxane bonds. These bonds are responsible for the compound’s ability to enhance adhesion and compatibility with various materials. Additionally, the amide group can interact with biological molecules through hydrogen bonding and electrostatic interactions, making it useful in bioconjugation and drug delivery applications.
相似化合物的比较
Similar Compounds
- N-(3-Triethoxysilylpropyl)gluconamide
- 3-(Triethoxysilyl)propyl isocyanate
- Bis[3-(triethoxysilyl)propyl]tetrasulfide
Uniqueness
N-(3-Triethoxysilylpropyl)-4-hydroxybutyramide is unique due to the presence of both silane and amide functional groups, which allows it to participate in a wide range of chemical reactions and form strong bonds with diverse substrates. This dual functionality makes it particularly valuable in applications requiring surface modification, bioconjugation, and the synthesis of hybrid materials.
生物活性
N-(3-Triethoxysilylpropyl)-4-hydroxybutyramide (TEOS-HB) is a silane compound with significant biological activity, particularly in bioconjugation and surface modification applications. Its unique structure combines a triethoxysilyl group with a 4-hydroxybutyramide moiety, allowing it to interact effectively with both organic and inorganic materials. This article explores the biological activity of TEOS-HB, including its mechanisms of action, applications, and relevant case studies.
Structural Characteristics
TEOS-HB has the molecular formula and a molecular weight of approximately 307.46 g/mol. The compound's structure facilitates its reactivity through two main functional groups:
- Silane Group : The triethoxysilyl group can form covalent bonds with inorganic surfaces such as glass or silicon dioxide (SiO₂).
- Amide Group : The 4-hydroxybutyramide moiety provides a reactive site for further attachment of biomolecules.
This dual functionality makes TEOS-HB an effective coupling agent in various chemical processes, particularly in the field of bioconjugation.
TEOS-HB exhibits biological activity through several mechanisms:
- Surface Functionalization : The silane group allows for the modification of surfaces to enhance adhesion and stability of biomolecules.
- Bioconjugation : The amide functionality enables the attachment of proteins, nucleic acids, and other biomolecules, facilitating their immobilization on surfaces.
- Controlled Release : TEOS-HB can be used to create systems for controlled release of therapeutic agents by modifying the surface properties of drug delivery vehicles.
Applications
TEOS-HB has diverse applications across various fields:
- Biomedical Devices : Used to enhance the biocompatibility and functionality of implants and biosensors.
- DNA Microarray Synthesis : Acts as an anchoring reagent for immobilizing DNA on glass slides, enabling high-throughput screening and analysis.
- Surface Coatings : Utilized in creating tailored substrates for research purposes, including studies on protein interactions and cellular responses.
Case Study 1: DNA Microarray Synthesis
In a study published in Nucleic Acids Research, TEOS-HB was employed to functionalize glass surfaces for DNA microarray synthesis. Researchers demonstrated that TEOS-HB could effectively immobilize photocleavable linker molecules, allowing for precise positioning of DNA strands during synthesis using light irradiation. This method significantly improved the efficiency and specificity of DNA microarrays (McGall et al., 1997) .
Case Study 2: Thrombin-Binding Aptamers
Another study highlighted the use of TEOS-HB in the development of G-quadruplex-based thrombin-binding aptamers. The functionalization of glass slides with TEOS-HB facilitated the immobilization of aptamers, which were then used to investigate their binding affinity to thrombin. The results indicated that TEOS-HB-modified surfaces provided enhanced stability and binding efficiency compared to unmodified surfaces (PMC5389548) .
Comparative Analysis with Similar Compounds
The following table summarizes some compounds that share structural similarities with TEOS-HB:
Compound Name | Structure Features | Unique Aspects |
---|---|---|
3-Aminopropyltriethoxysilane | Contains an amine group instead of an amide | Primarily used for adhesion promotion |
3-Mercaptopropyltriethoxysilane | Contains a thiol group | Useful in creating metal nanoparticle composites |
4-Hydroxybutyric acid | Lacks the silane functionality | Primarily used in pharmaceutical applications |
TEOS-HB stands out due to its dual functionality, allowing effective interaction with both organic and inorganic materials.
属性
IUPAC Name |
4-hydroxy-N-(3-triethoxysilylpropyl)butanamide | |
---|---|---|
Source | PubChem | |
URL | https://pubchem.ncbi.nlm.nih.gov | |
Description | Data deposited in or computed by PubChem | |
InChI |
InChI=1S/C13H29NO5Si/c1-4-17-20(18-5-2,19-6-3)12-8-10-14-13(16)9-7-11-15/h15H,4-12H2,1-3H3,(H,14,16) | |
Source | PubChem | |
URL | https://pubchem.ncbi.nlm.nih.gov | |
Description | Data deposited in or computed by PubChem | |
InChI Key |
QKDAMFXBOUOVMF-UHFFFAOYSA-N | |
Source | PubChem | |
URL | https://pubchem.ncbi.nlm.nih.gov | |
Description | Data deposited in or computed by PubChem | |
Canonical SMILES |
CCO[Si](CCCNC(=O)CCCO)(OCC)OCC | |
Source | PubChem | |
URL | https://pubchem.ncbi.nlm.nih.gov | |
Description | Data deposited in or computed by PubChem | |
Molecular Formula |
C13H29NO5Si | |
Source | PubChem | |
URL | https://pubchem.ncbi.nlm.nih.gov | |
Description | Data deposited in or computed by PubChem | |
Molecular Weight |
307.46 g/mol | |
Source | PubChem | |
URL | https://pubchem.ncbi.nlm.nih.gov | |
Description | Data deposited in or computed by PubChem | |
CAS No. |
186543-03-3 | |
Record name | N-(3-Triethoxysilylpropyl)-4-hydroxybutyramide | |
Source | European Chemicals Agency (ECHA) | |
URL | https://echa.europa.eu/information-on-chemicals | |
Description | The European Chemicals Agency (ECHA) is an agency of the European Union which is the driving force among regulatory authorities in implementing the EU's groundbreaking chemicals legislation for the benefit of human health and the environment as well as for innovation and competitiveness. | |
Explanation | Use of the information, documents and data from the ECHA website is subject to the terms and conditions of this Legal Notice, and subject to other binding limitations provided for under applicable law, the information, documents and data made available on the ECHA website may be reproduced, distributed and/or used, totally or in part, for non-commercial purposes provided that ECHA is acknowledged as the source: "Source: European Chemicals Agency, http://echa.europa.eu/". Such acknowledgement must be included in each copy of the material. ECHA permits and encourages organisations and individuals to create links to the ECHA website under the following cumulative conditions: Links can only be made to webpages that provide a link to the Legal Notice page. | |
Retrosynthesis Analysis
AI-Powered Synthesis Planning: Our tool employs the Template_relevance Pistachio, Template_relevance Bkms_metabolic, Template_relevance Pistachio_ringbreaker, Template_relevance Reaxys, Template_relevance Reaxys_biocatalysis model, leveraging a vast database of chemical reactions to predict feasible synthetic routes.
One-Step Synthesis Focus: Specifically designed for one-step synthesis, it provides concise and direct routes for your target compounds, streamlining the synthesis process.
Accurate Predictions: Utilizing the extensive PISTACHIO, BKMS_METABOLIC, PISTACHIO_RINGBREAKER, REAXYS, REAXYS_BIOCATALYSIS database, our tool offers high-accuracy predictions, reflecting the latest in chemical research and data.
Strategy Settings
Precursor scoring | Relevance Heuristic |
---|---|
Min. plausibility | 0.01 |
Model | Template_relevance |
Template Set | Pistachio/Bkms_metabolic/Pistachio_ringbreaker/Reaxys/Reaxys_biocatalysis |
Top-N result to add to graph | 6 |
Feasible Synthetic Routes
体外研究产品的免责声明和信息
请注意,BenchChem 上展示的所有文章和产品信息仅供信息参考。 BenchChem 上可购买的产品专为体外研究设计,这些研究在生物体外进行。体外研究,源自拉丁语 "in glass",涉及在受控实验室环境中使用细胞或组织进行的实验。重要的是要注意,这些产品没有被归类为药物或药品,他们没有得到 FDA 的批准,用于预防、治疗或治愈任何医疗状况、疾病或疾病。我们必须强调,将这些产品以任何形式引入人类或动物的身体都是法律严格禁止的。遵守这些指南对确保研究和实验的法律和道德标准的符合性至关重要。