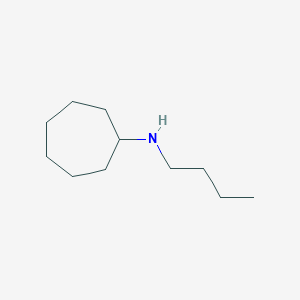
N-丁基环庚胺
描述
N-butylcycloheptanamine is a chemical compound with the molecular formula C11H23N . It is also known as N-butyl-N-cycloheptylamine . The hydrochloride salt of N-butylcycloheptanamine has a CAS Number of 1609407-31-9 .
Synthesis Analysis
The synthesis of amines like N-butylcycloheptanamine can be achieved through various methods. One common method is the reduction of nitriles or amides . Another method involves the alkylation of potassium phthalimide, followed by hydrolysis of the N-alkyl phthalimide . These methods are part of a larger set of reactions involving alkyl groups, such as SN2 reactions of alkyl halides, ammonia, and other amines .Molecular Structure Analysis
The molecular structure of N-butylcycloheptanamine can be represented by the InChI code: 1S/C11H23N.ClH/c1-2-3-10-12-11-8-6-4-5-7-9-11;/h11-12H,2-10H2,1H3;1H . This indicates that the molecule consists of a cycloheptanamine group with a butyl group attached to the nitrogen atom .Physical And Chemical Properties Analysis
N-butylcycloheptanamine has a molecular weight of 205.77 . It is a solid at room temperature . The compound has a density of 0.84±0.1 g/cm3 .科学研究应用
芳基环己胺的分析特性:芳基环己胺,包括与N-丁基环庚胺在结构上相关的物质,使用各种分析方法进行了表征。这些化合物通常被宣传为“研究化学品”,已经通过气相色谱和液相色谱-质谱等方法研究其在血液、尿液和玻璃体液等生物基质中的存在 (De Paoli, Brandt, Wallach, Archer, & Pounder, 2013)。
合成和分析表征:研究重点放在合成各种N-烷基-芳基环己胺,与N-丁基环庚胺相关的化合物,并使用不同技术对其进行分析。这些化合物被认为是类似氯胺酮的解离性物质,据信主要通过拮抗N-甲基-D-天冬氨酸(NMDA)受体发挥作用 (Wallach et al., 2016)。
重症监护中中毒案例分析:一项案例研究详细描述了一名男子因芳基环己胺化合物中毒,展示了分子网络在识别和处理新型致幻物质(NPS)案例中的应用 (Pelletier et al., 2021)。
谷胱甘肽合成的抑制:已经对像丁硫胺氧化亚胺这样的化合物进行了研究,它们在结构上与N-丁基环庚胺相关,具有强大和特异的抑制谷胱甘肽合成的作用。这对生物学中的各种实验系统具有重要意义 (Griffith & Meister, 1979)。
新化合物的细胞毒作用:一项关于合成和表征环己基1,3-丙二胺-N,N'-二乙酸盐分子的研究,与N-丁基环庚胺相关,显示出强大的抗肿瘤活性,特别是对人类胶质瘤细胞 (Savić等,2014)。
特定吸入挑战的方法学:涉及N-丁基醋酸酯,一种与N-丁基环庚胺相关的化合物,用于设计通过吸入挑战诊断职业性哮喘(OA)的设备 (Caron, Boileau, Malo, & Leblond, 2010)。
安全和危害
属性
IUPAC Name |
N-butylcycloheptanamine | |
---|---|---|
Source | PubChem | |
URL | https://pubchem.ncbi.nlm.nih.gov | |
Description | Data deposited in or computed by PubChem | |
InChI |
InChI=1S/C11H23N/c1-2-3-10-12-11-8-6-4-5-7-9-11/h11-12H,2-10H2,1H3 | |
Source | PubChem | |
URL | https://pubchem.ncbi.nlm.nih.gov | |
Description | Data deposited in or computed by PubChem | |
InChI Key |
UAEMGQGRVOWQKX-UHFFFAOYSA-N | |
Source | PubChem | |
URL | https://pubchem.ncbi.nlm.nih.gov | |
Description | Data deposited in or computed by PubChem | |
Canonical SMILES |
CCCCNC1CCCCCC1 | |
Source | PubChem | |
URL | https://pubchem.ncbi.nlm.nih.gov | |
Description | Data deposited in or computed by PubChem | |
Molecular Formula |
C11H23N | |
Source | PubChem | |
URL | https://pubchem.ncbi.nlm.nih.gov | |
Description | Data deposited in or computed by PubChem | |
DSSTOX Substance ID |
DTXSID50440659 | |
Record name | N-butylcycloheptanamine | |
Source | EPA DSSTox | |
URL | https://comptox.epa.gov/dashboard/DTXSID50440659 | |
Description | DSSTox provides a high quality public chemistry resource for supporting improved predictive toxicology. | |
Molecular Weight |
169.31 g/mol | |
Source | PubChem | |
URL | https://pubchem.ncbi.nlm.nih.gov | |
Description | Data deposited in or computed by PubChem | |
Product Name |
N-butylcycloheptanamine | |
CAS RN |
161012-67-5 | |
Record name | N-butylcycloheptanamine | |
Source | EPA DSSTox | |
URL | https://comptox.epa.gov/dashboard/DTXSID50440659 | |
Description | DSSTox provides a high quality public chemistry resource for supporting improved predictive toxicology. | |
Retrosynthesis Analysis
AI-Powered Synthesis Planning: Our tool employs the Template_relevance Pistachio, Template_relevance Bkms_metabolic, Template_relevance Pistachio_ringbreaker, Template_relevance Reaxys, Template_relevance Reaxys_biocatalysis model, leveraging a vast database of chemical reactions to predict feasible synthetic routes.
One-Step Synthesis Focus: Specifically designed for one-step synthesis, it provides concise and direct routes for your target compounds, streamlining the synthesis process.
Accurate Predictions: Utilizing the extensive PISTACHIO, BKMS_METABOLIC, PISTACHIO_RINGBREAKER, REAXYS, REAXYS_BIOCATALYSIS database, our tool offers high-accuracy predictions, reflecting the latest in chemical research and data.
Strategy Settings
Precursor scoring | Relevance Heuristic |
---|---|
Min. plausibility | 0.01 |
Model | Template_relevance |
Template Set | Pistachio/Bkms_metabolic/Pistachio_ringbreaker/Reaxys/Reaxys_biocatalysis |
Top-N result to add to graph | 6 |
Feasible Synthetic Routes
体外研究产品的免责声明和信息
请注意,BenchChem 上展示的所有文章和产品信息仅供信息参考。 BenchChem 上可购买的产品专为体外研究设计,这些研究在生物体外进行。体外研究,源自拉丁语 "in glass",涉及在受控实验室环境中使用细胞或组织进行的实验。重要的是要注意,这些产品没有被归类为药物或药品,他们没有得到 FDA 的批准,用于预防、治疗或治愈任何医疗状况、疾病或疾病。我们必须强调,将这些产品以任何形式引入人类或动物的身体都是法律严格禁止的。遵守这些指南对确保研究和实验的法律和道德标准的符合性至关重要。