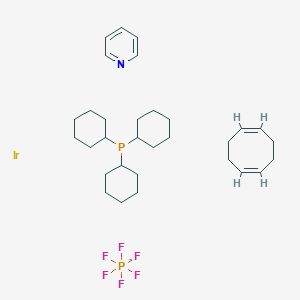
Crabtree's catalyst
描述
Crabtree's catalyst, also known as this compound, is a useful research compound. Its molecular formula is C31H50F6IrNP2- and its molecular weight is 804.9 g/mol. The purity is usually 95%.
The exact mass of the compound this compound is unknown and the complexity rating of the compound is unknown. The United Nations designated GHS hazard class pictogram is Irritant, and the GHS signal word is WarningThe storage condition is unknown. Please store according to label instructions upon receipt of goods.
BenchChem offers high-quality this compound suitable for many research applications. Different packaging options are available to accommodate customers' requirements. Please inquire for more information about this compound including the price, delivery time, and more detailed information at info@benchchem.com.
科学研究应用
均相氢化
Crabtree's 催化剂,一种基于铱的化合物,于 1977 年问世,标志着均相催化和烯烃催化氢化的范式转变 . 它具有很高的活性,可以催化四取代烯烃的氢化,这对于其他催化剂来说通常很困难 .
不对称氢化
1998 年,Pfaltz 使用 BARF 作为阴离子,引入了一种改进的催化剂,并建立了第一个 Crabtree 催化剂的手性变体 . 这导致了大量实用性高且对映选择性高的合成方法 . 源自 Crabtree 催化剂的手性铱配合物在现代烯烃氢化中非常有用,这归因于其高反应性、立体选择性和稳定性 .
同位素交换反应
Crabtree's 催化剂用于同位素交换反应。 更具体地说,它催化氢原子与其同位素氘和氚之间的直接交换,而无需使用中间体 . 研究表明,Crabtree's 催化剂的同位素交换具有高度的区域选择性 .
机理研究
由于关键中间体的稳定性问题,阐明机理细节带来了很大的困难。Brandt、Andersson 等人取得了重大突破。 2003 年,基于 DFT 计算 . 这些作者用一个新的 IrIII/IrV 循环取代了之前假设的 IrI/IrIII 催化循环 .
非均相氢化
Crabtree's 催化剂通过阳离子交换被封装在磺化 MIL-101 (Cr) 金属有机框架 (MOF) 的孔隙中。 这种杂化催化剂对非官能化烯烃的非均相氢化具有活性,无论是在溶液中还是在气相中
作用机制
Target of Action
Crabtree’s catalyst is an organoiridium compound with the formula [C₈H₁₂IrP(C₆H₁₁)₃C₅H₅N]PF₆. Its primary targets are olefinic substrates, specifically tetrasubstituted olefins. Unlike other catalysts (such as Wilkinson’s catalyst), Crabtree’s catalyst can effectively hydrogenate these challenging substrates .
Mode of Action
The complex operates via an intermediate, such as cis-[IrH₂(cod)L₂] (cationic charge not shown). It exhibits square planar molecular geometry due to its d⁸ electronic configuration. The hydrogenation reaction occurs at room temperature without meticulous solvent drying or deoxygenation. Crabtree’s catalyst is sensitive to proton-bearing impurities and becomes irreversibly deactivated after about ten minutes, indicated by a yellow color. This deactivation process involves the formation of hydride-bridged dimers .
Biochemical Pathways
Crabtree’s catalyst selectively hydrogenates tetrasubstituted olefins, even in the presence of coordinating functional groups. Its unique ability to target these substrates makes it a valuable tool in synthetic chemistry. The distribution of isomers shifts in favor of the cis isomer when the catalyst operates in dichloromethane, thanks to a bonding interaction between the hydroxyl group and the iridium center .
Action Environment
Environmental factors, such as solvent choice and temperature, influence the catalyst’s efficacy and stability. Researchers must consider these conditions to optimize its performance.
生化分析
Biochemical Properties
Crabtree’s catalyst is effective for the hydrogenations of mono-, di-, tri-, and tetra-substituted substrates . It is known for its directed hydrogenation to give trans stereoselectivity
Molecular Mechanism
Crabtree’s catalyst operates via an intermediate such as cis - [IrH2(cod)L2] (cationic charge not shown) . It is reactive at room temperature and is tolerant of weakly basic functional groups . The catalyst becomes irreversibly deactivated after about ten minutes at room temperature .
Temporal Effects in Laboratory Settings
The extent of time-dependent deactivation of Crabtree’s catalyst by trimer formation is strongly dependent on ligand structure . One deactivation process involves the formation of hydride-bridged dimers .
属性
IUPAC Name |
cycloocta-1,5-diene;iridium;pyridine;tricyclohexylphosphane;hexafluorophosphate | |
---|---|---|
Details | Computed by Lexichem TK 2.7.0 (PubChem release 2021.05.07) | |
Source | PubChem | |
URL | https://pubchem.ncbi.nlm.nih.gov | |
Description | Data deposited in or computed by PubChem | |
InChI |
InChI=1S/C18H33P.C8H12.C5H5N.F6P.Ir/c1-4-10-16(11-5-1)19(17-12-6-2-7-13-17)18-14-8-3-9-15-18;1-2-4-6-8-7-5-3-1;1-2-4-6-5-3-1;1-7(2,3,4,5)6;/h16-18H,1-15H2;1-2,7-8H,3-6H2;1-5H;;/q;;;-1; | |
Details | Computed by InChI 1.0.6 (PubChem release 2021.05.07) | |
Source | PubChem | |
URL | https://pubchem.ncbi.nlm.nih.gov | |
Description | Data deposited in or computed by PubChem | |
InChI Key |
WLRQNTYCIFESRH-UHFFFAOYSA-N | |
Details | Computed by InChI 1.0.6 (PubChem release 2021.05.07) | |
Source | PubChem | |
URL | https://pubchem.ncbi.nlm.nih.gov | |
Description | Data deposited in or computed by PubChem | |
Canonical SMILES |
C1CCC(CC1)P(C2CCCCC2)C3CCCCC3.C1CC=CCCC=C1.C1=CC=NC=C1.F[P-](F)(F)(F)(F)F.[Ir] | |
Details | Computed by OEChem 2.3.0 (PubChem release 2021.05.07) | |
Source | PubChem | |
URL | https://pubchem.ncbi.nlm.nih.gov | |
Description | Data deposited in or computed by PubChem | |
Molecular Formula |
C31H50F6IrNP2- | |
Details | Computed by PubChem 2.1 (PubChem release 2021.05.07) | |
Source | PubChem | |
URL | https://pubchem.ncbi.nlm.nih.gov | |
Description | Data deposited in or computed by PubChem | |
Molecular Weight |
804.9 g/mol | |
Details | Computed by PubChem 2.1 (PubChem release 2021.05.07) | |
Source | PubChem | |
URL | https://pubchem.ncbi.nlm.nih.gov | |
Description | Data deposited in or computed by PubChem | |
CAS No. |
64536-78-3 | |
Record name | (Tricyclohexylphosphine)(1,5-cyclooctadiene)(pyridine)iridium(I) hexafluorophosphate | |
Source | European Chemicals Agency (ECHA) | |
URL | https://echa.europa.eu/information-on-chemicals | |
Description | The European Chemicals Agency (ECHA) is an agency of the European Union which is the driving force among regulatory authorities in implementing the EU's groundbreaking chemicals legislation for the benefit of human health and the environment as well as for innovation and competitiveness. | |
Explanation | Use of the information, documents and data from the ECHA website is subject to the terms and conditions of this Legal Notice, and subject to other binding limitations provided for under applicable law, the information, documents and data made available on the ECHA website may be reproduced, distributed and/or used, totally or in part, for non-commercial purposes provided that ECHA is acknowledged as the source: "Source: European Chemicals Agency, http://echa.europa.eu/". Such acknowledgement must be included in each copy of the material. ECHA permits and encourages organisations and individuals to create links to the ECHA website under the following cumulative conditions: Links can only be made to webpages that provide a link to the Legal Notice page. | |
Retrosynthesis Analysis
AI-Powered Synthesis Planning: Our tool employs the Template_relevance Pistachio, Template_relevance Bkms_metabolic, Template_relevance Pistachio_ringbreaker, Template_relevance Reaxys, Template_relevance Reaxys_biocatalysis model, leveraging a vast database of chemical reactions to predict feasible synthetic routes.
One-Step Synthesis Focus: Specifically designed for one-step synthesis, it provides concise and direct routes for your target compounds, streamlining the synthesis process.
Accurate Predictions: Utilizing the extensive PISTACHIO, BKMS_METABOLIC, PISTACHIO_RINGBREAKER, REAXYS, REAXYS_BIOCATALYSIS database, our tool offers high-accuracy predictions, reflecting the latest in chemical research and data.
Strategy Settings
Precursor scoring | Relevance Heuristic |
---|---|
Min. plausibility | 0.01 |
Model | Template_relevance |
Template Set | Pistachio/Bkms_metabolic/Pistachio_ringbreaker/Reaxys/Reaxys_biocatalysis |
Top-N result to add to graph | 6 |
Feasible Synthetic Routes
体外研究产品的免责声明和信息
请注意,BenchChem 上展示的所有文章和产品信息仅供信息参考。 BenchChem 上可购买的产品专为体外研究设计,这些研究在生物体外进行。体外研究,源自拉丁语 "in glass",涉及在受控实验室环境中使用细胞或组织进行的实验。重要的是要注意,这些产品没有被归类为药物或药品,他们没有得到 FDA 的批准,用于预防、治疗或治愈任何医疗状况、疾病或疾病。我们必须强调,将这些产品以任何形式引入人类或动物的身体都是法律严格禁止的。遵守这些指南对确保研究和实验的法律和道德标准的符合性至关重要。