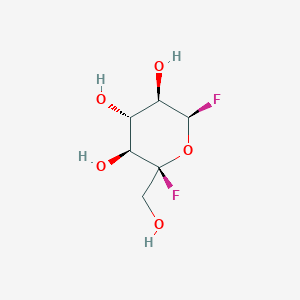
5-Fluoro-alpha-d-glucopyranosyl fluoride
- 点击 快速询问 获取最新报价。
- 提供有竞争力价格的高质量产品,您可以更专注于研究。
描述
5-Fluoro-alpha-d-glucopyranosyl fluoride is a fluorinated carbohydrate derivative. It is a synthetic compound that has garnered interest due to its unique chemical properties and potential applications in various scientific fields. The compound is characterized by the presence of a fluorine atom at the 5-position of the glucopyranosyl ring, which significantly alters its chemical behavior compared to non-fluorinated analogs.
准备方法
Synthetic Routes and Reaction Conditions
The synthesis of 5-Fluoro-alpha-d-glucopyranosyl fluoride typically involves the fluorination of a suitable glucopyranosyl precursor. One common method is the reaction of alpha-d-glucopyranosyl fluoride with a fluorinating agent such as diethylaminosulfur trifluoride (DAST) or xenon difluoride (XeF2). The reaction is usually carried out in an anhydrous solvent like dichloromethane or acetonitrile, under controlled temperature conditions to prevent decomposition .
Industrial Production Methods
Industrial production of this compound follows similar synthetic routes but on a larger scale. The process involves the use of high-purity reagents and advanced fluorination techniques to ensure the consistency and quality of the final product. The reaction conditions are optimized to maximize yield and minimize by-products, often involving continuous flow reactors and automated systems for precise control .
化学反应分析
Types of Reactions
5-Fluoro-alpha-d-glucopyranosyl fluoride undergoes several types of chemical reactions, including:
Hydrolysis: Catalyzed by glycoside hydrolases, leading to the formation of alpha-d-glucopyranose and fluoride ion.
Substitution: The fluorine atom can be substituted by other nucleophiles under appropriate conditions.
Oxidation and Reduction: The compound can participate in redox reactions, although these are less common due to the stability of the fluorine-carbon bond.
Common Reagents and Conditions
Hydrolysis: Enzymatic hydrolysis using glycoside hydrolases such as beta-glucosidase.
Substitution: Nucleophilic substitution reactions using reagents like sodium azide or thiols.
Oxidation and Reduction: Catalysts such as palladium on carbon (Pd/C) for hydrogenation reactions.
Major Products Formed
Hydrolysis: Alpha-d-glucopyranose and fluoride ion.
Substitution: Various substituted glucopyranosyl derivatives depending on the nucleophile used.
Oxidation and Reduction: Reduced or oxidized forms of the glucopyranosyl ring.
科学研究应用
Chemistry
5F-α-GlcF serves as a model compound in carbohydrate chemistry to study the effects of fluorination. Its unique properties allow researchers to investigate reaction mechanisms and the influence of fluorine on carbohydrate reactivity.
Key Reactions:
- Hydrolysis: Catalyzed by glycoside hydrolases, resulting in alpha-d-glucopyranose and fluoride ion.
- Substitution: The fluorine atom can be substituted by nucleophiles, leading to various derivatives.
- Oxidation/Reduction: Participates in redox reactions, although these are less common due to the stability of the fluorine-carbon bond.
Biology
In biological research, 5F-α-GlcF is utilized to probe enzyme mechanisms, particularly those involving glycoside hydrolases. It acts as a substrate that undergoes hydrolysis, providing insights into enzyme kinetics and substrate specificity.
Case Study:
A study demonstrated the use of 5F-α-GlcF as a mechanistic probe for Escherichia coli β-glucuronidase, where it successfully trapped a glycosyl-enzyme intermediate, allowing for detailed analysis of enzyme activity .
Industry
In industrial applications, 5F-α-GlcF is employed in the synthesis of specialty chemicals and as a precursor for more complex fluorinated compounds. Its unique properties make it valuable in developing new materials with enhanced performance characteristics.
作用机制
The mechanism of action of 5-Fluoro-alpha-d-glucopyranosyl fluoride primarily involves its interaction with glycoside hydrolases. The compound acts as a substrate for these enzymes, undergoing hydrolysis to release alpha-d-glucopyranose and fluoride ion. The presence of the fluorine atom at the 5-position influences the enzyme’s catalytic activity and provides insights into the enzyme’s mechanism of action . The molecular targets include the active sites of glycoside hydrolases, where the compound forms a glycosyl-enzyme intermediate .
相似化合物的比较
Similar Compounds
Alpha-d-glucopyranosyl fluoride: Lacks the fluorine atom at the 5-position.
1-Fluoro-1-deoxy-alpha-d-glucose: Fluorine atom at the 1-position instead of the 5-position.
2-Fluoro-2-deoxy-d-glucose: Fluorine atom at the 2-position.
Uniqueness
5-Fluoro-alpha-d-glucopyranosyl fluoride is unique due to the specific positioning of the fluorine atom at the 5-position, which significantly alters its chemical and enzymatic properties compared to other fluorinated glucose derivatives. This unique positioning makes it a valuable tool for studying enzyme mechanisms and developing fluorinated analogs for various applications .
生物活性
5-Fluoro-alpha-d-glucopyranosyl fluoride (5-F-α-D-GluF) is a fluorinated carbohydrate derivative that has attracted considerable attention in biochemical research due to its unique structural properties and biological activity. This article provides a comprehensive overview of its biological activity, including synthesis methods, mechanisms of action, and applications in various fields.
Chemical Structure and Synthesis
5-F-α-D-GluF is characterized by the presence of a fluorine atom at the 5-position of the glucopyranosyl ring. This modification significantly alters its chemical behavior compared to non-fluorinated analogs. The synthesis typically involves the fluorination of a suitable glucopyranosyl precursor using reagents such as diethylaminosulfur trifluoride (DAST) or xenon difluoride (XeF2) in anhydrous solvents like dichloromethane or acetonitrile .
Synthesis Overview
Method | Reagents | Conditions |
---|---|---|
Fluorination | DAST, XeF2 | Anhydrous solvent, controlled temperature |
Hydrolysis | Glycoside hydrolases (e.g., β-glucosidase) | Enzymatic conditions |
Substitution | Nucleophiles (e.g., sodium azide) | Variable conditions |
The primary biological activity of 5-F-α-D-GluF is its interaction with glycoside hydrolases, where it acts as a substrate. The hydrolysis of this compound results in the release of alpha-d-glucopyranose and fluoride ion. The fluorine atom's presence at the 5-position influences enzyme kinetics, providing insights into enzyme mechanisms and potential applications in drug design .
Enzymatic Interactions
- Hydrolysis : Catalyzed by glycoside hydrolases, leading to the production of alpha-d-glucopyranose.
- Substitution Reactions : The fluorine can be replaced by other nucleophiles under specific conditions.
- Inhibition Studies : 5-F-α-D-GluF has been used as an inhibitor for various glycosidases, showcasing its potential in drug discovery .
Biological Activity and Applications
The biological activity of 5-F-α-D-GluF is notable for its ability to mimic natural sugars while exhibiting distinct properties due to fluorination. It has been studied for various applications:
- Enzyme Mechanism Studies : Used to investigate the catalytic mechanisms of glycoside hydrolases.
- Drug Development : Its structural properties allow it to serve as a lead compound for designing glycomimetics that can resist enzymatic degradation.
- Toxicity Assessment : Preliminary studies indicate that it may exhibit cytotoxic effects, making it relevant for cancer research .
Case Study 1: Inhibition of Glycosidases
In a study examining the inhibition of α-glycosidase enzymes, 5-F-α-D-GluF was shown to effectively inhibit enzyme activity, suggesting its potential as a therapeutic agent for managing conditions like diabetes .
Case Study 2: Fluorinated Glycomimetics
Research highlighted the synthesis of fluorinated glycomimetics based on 5-F-α-D-GluF, demonstrating enhanced stability and resistance to hydrolysis compared to their non-fluorinated counterparts. These compounds exhibited improved bioavailability and selectivity for target enzymes .
Comparative Analysis with Similar Compounds
The unique positioning of the fluorine atom at the 5-position distinguishes 5-F-α-D-GluF from other fluorinated carbohydrates:
Compound | Fluorine Position | Biological Activity |
---|---|---|
Alpha-d-glucopyranosyl fluoride | None | Natural substrate for glycosidases |
1-Fluoro-1-deoxy-alpha-D-glucose | C-1 | Different enzymatic interactions |
2-Fluoro-2-deoxy-D-glucose | C-2 | Altered metabolic pathways |
属性
IUPAC Name |
(2S,3S,4R,5R,6R)-2,6-difluoro-2-(hydroxymethyl)oxane-3,4,5-triol |
Source
|
---|---|---|
Source | PubChem | |
URL | https://pubchem.ncbi.nlm.nih.gov | |
Description | Data deposited in or computed by PubChem | |
InChI |
InChI=1S/C6H10F2O5/c7-5-3(11)2(10)4(12)6(8,1-9)13-5/h2-5,9-12H,1H2/t2-,3-,4+,5+,6-/m1/s1 |
Source
|
Source | PubChem | |
URL | https://pubchem.ncbi.nlm.nih.gov | |
Description | Data deposited in or computed by PubChem | |
InChI Key |
MGHYRMVVRYCAON-RWOPYEJCSA-N |
Source
|
Source | PubChem | |
URL | https://pubchem.ncbi.nlm.nih.gov | |
Description | Data deposited in or computed by PubChem | |
Canonical SMILES |
C(C1(C(C(C(C(O1)F)O)O)O)F)O |
Source
|
Source | PubChem | |
URL | https://pubchem.ncbi.nlm.nih.gov | |
Description | Data deposited in or computed by PubChem | |
Isomeric SMILES |
C([C@@]1([C@H]([C@@H]([C@H]([C@H](O1)F)O)O)O)F)O |
Source
|
Source | PubChem | |
URL | https://pubchem.ncbi.nlm.nih.gov | |
Description | Data deposited in or computed by PubChem | |
Molecular Formula |
C6H10F2O5 |
Source
|
Source | PubChem | |
URL | https://pubchem.ncbi.nlm.nih.gov | |
Description | Data deposited in or computed by PubChem | |
Molecular Weight |
200.14 g/mol |
Source
|
Source | PubChem | |
URL | https://pubchem.ncbi.nlm.nih.gov | |
Description | Data deposited in or computed by PubChem | |
体外研究产品的免责声明和信息
请注意,BenchChem 上展示的所有文章和产品信息仅供信息参考。 BenchChem 上可购买的产品专为体外研究设计,这些研究在生物体外进行。体外研究,源自拉丁语 "in glass",涉及在受控实验室环境中使用细胞或组织进行的实验。重要的是要注意,这些产品没有被归类为药物或药品,他们没有得到 FDA 的批准,用于预防、治疗或治愈任何医疗状况、疾病或疾病。我们必须强调,将这些产品以任何形式引入人类或动物的身体都是法律严格禁止的。遵守这些指南对确保研究和实验的法律和道德标准的符合性至关重要。