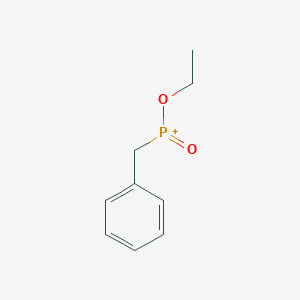
Benzyl(ethoxy)oxophosphanium
- 点击 快速询问 获取最新报价。
- 提供有竞争力价格的高质量产品,您可以更专注于研究。
描述
Benzyl(ethoxy)oxophosphanium is an organophosphorus compound characterized by the presence of a benzyl group, an ethoxy group, and an oxophosphanium moiety
准备方法
Synthetic Routes and Reaction Conditions: Benzyl(ethoxy)oxophosphanium can be synthesized through a multi-step process involving the reaction of benzyl chloride with triethyl phosphite, followed by oxidation. The reaction typically proceeds under mild conditions, with the use of a suitable solvent such as dichloromethane. The general reaction scheme is as follows: [ \text{C}_6\text{H}_5\text{CH}_2\text{Cl} + \text{(EtO)}_3\text{P} \rightarrow \text{C}_6\text{H}_5\text{CH}_2\text{P(OEt)}_2 ] [ \text{C}_6\text{H}_5\text{CH}_2\text{P(OEt)}_2 + \text{Oxidizing Agent} \rightarrow \text{C}_6\text{H}_5\text{CH}_2\text{P(OEt)}_2\text{O} ]
Industrial Production Methods: Industrial production of this compound involves similar synthetic routes but on a larger scale. The process is optimized for higher yields and purity, often employing continuous flow reactors and advanced purification techniques.
Types of Reactions:
Oxidation: this compound can undergo oxidation reactions, typically forming phosphine oxides.
Reduction: It can be reduced to form phosphines.
Substitution: The compound can participate in nucleophilic substitution reactions, where the ethoxy group can be replaced by other nucleophiles.
Common Reagents and Conditions:
Oxidation: Common oxidizing agents include hydrogen peroxide and m-chloroperbenzoic acid.
Reduction: Reducing agents such as lithium aluminum hydride are used.
Substitution: Nucleophiles like amines or thiols can be used under basic conditions.
Major Products:
Oxidation: Phosphine oxides.
Reduction: Phosphines.
Substitution: Various substituted phosphonium salts.
科学研究应用
Benzyl(ethoxy)oxophosphanium has several applications in scientific research:
Chemistry: It is used as a reagent in organic synthesis, particularly in the formation of carbon-phosphorus bonds.
Biology: The compound is studied for its potential role in biochemical pathways involving phosphorus-containing compounds.
Medicine: Research is ongoing into its potential use as a precursor for pharmaceuticals.
Industry: It is used in the production of flame retardants and plasticizers.
作用机制
The mechanism by which Benzyl(ethoxy)oxophosphanium exerts its effects involves the interaction of the phosphonium moiety with various molecular targets. The compound can act as a nucleophile or electrophile, depending on the reaction conditions. It is involved in pathways that include the formation and cleavage of phosphorus-oxygen and phosphorus-carbon bonds.
相似化合物的比较
Triphenylphosphine: Similar in that it contains a phosphorus atom bonded to aromatic groups.
Diethylphosphite: Contains ethoxy groups bonded to phosphorus.
Phosphine Oxides: Share the phosphine oxide functional group.
生物活性
Benzyl(ethoxy)oxophosphanium, an organophosphorus compound, has garnered attention in various fields, including medicinal chemistry and organic synthesis. Its unique structure, characterized by a benzyl group, an ethoxy group, and an oxophosphanium moiety, allows it to engage in diverse biological activities. This article provides a comprehensive overview of its biological activity, including mechanisms of action, applications in research, and case studies highlighting its significance.
Chemical Structure and Synthesis
This compound can be synthesized through a multi-step process involving the reaction of benzyl chloride with triethyl phosphite, followed by oxidation. The general reaction scheme is as follows:
This synthesis typically occurs under mild conditions using solvents like dichloromethane. The compound's ability to undergo oxidation and reduction reactions makes it a versatile reagent in organic synthesis.
The biological activity of this compound is largely attributed to its interaction with various molecular targets. The phosphonium moiety can act as both a nucleophile and an electrophile, participating in biochemical pathways that involve the formation and cleavage of phosphorus-oxygen and phosphorus-carbon bonds. This dual functionality allows the compound to influence several physiological processes.
Medicinal Chemistry
Research into this compound indicates its potential as a precursor for pharmaceuticals. Its role in biochemical pathways involving phosphorus-containing compounds suggests applications in drug design and development. For instance, it may serve as a building block for synthesizing novel therapeutic agents targeting specific diseases.
Chemical Research
In organic synthesis, this compound is utilized for forming carbon-phosphorus bonds. Its reactivity enables the preparation of various phosphorus-containing compounds, which are essential in materials science and medicinal chemistry.
Case Study 1: Antidiabetic Drug Development
A relevant case study explored the synthesis of thiazolidinedione derivatives that activate peroxisome proliferator-activated receptor gamma (PPARγ), which enhances insulin sensitivity. Researchers have investigated similar organophosphorus compounds to evaluate their efficacy in managing diabetes. This compound's structural characteristics may provide insights into developing effective antidiabetic agents .
Case Study 2: Structure-Based Drug Design
Another study focused on structure-based drug design methodologies that leverage compounds like this compound for therapeutic applications. By understanding the interaction between this compound and biological targets, researchers can optimize drug candidates for diseases such as Alzheimer's .
Comparative Analysis with Similar Compounds
Compound | Structure | Biological Activity |
---|---|---|
This compound | Benzyl group + Ethoxy + Phosphonium moiety | Potential pharmaceutical precursor |
Triphenylphosphine | Phosphorus bonded to three phenyl groups | Used in organic synthesis |
Diethylphosphite | Ethoxy groups bonded to phosphorus | Versatile reagent in organic synthesis |
Phosphine Oxides | Oxidized phosphines | Involved in various chemical reactions |
属性
IUPAC Name |
benzyl-ethoxy-oxophosphanium |
Source
|
---|---|---|
Source | PubChem | |
URL | https://pubchem.ncbi.nlm.nih.gov | |
Description | Data deposited in or computed by PubChem | |
InChI |
InChI=1S/C9H12O2P/c1-2-11-12(10)8-9-6-4-3-5-7-9/h3-7H,2,8H2,1H3/q+1 |
Source
|
Source | PubChem | |
URL | https://pubchem.ncbi.nlm.nih.gov | |
Description | Data deposited in or computed by PubChem | |
InChI Key |
VKDFMKUOEXNCBQ-UHFFFAOYSA-N |
Source
|
Source | PubChem | |
URL | https://pubchem.ncbi.nlm.nih.gov | |
Description | Data deposited in or computed by PubChem | |
Canonical SMILES |
CCO[P+](=O)CC1=CC=CC=C1 |
Source
|
Source | PubChem | |
URL | https://pubchem.ncbi.nlm.nih.gov | |
Description | Data deposited in or computed by PubChem | |
Molecular Formula |
C9H12O2P+ |
Source
|
Source | PubChem | |
URL | https://pubchem.ncbi.nlm.nih.gov | |
Description | Data deposited in or computed by PubChem | |
DSSTOX Substance ID |
DTXSID20433730 |
Source
|
Record name | Benzyl(ethoxy)oxophosphanium | |
Source | EPA DSSTox | |
URL | https://comptox.epa.gov/dashboard/DTXSID20433730 | |
Description | DSSTox provides a high quality public chemistry resource for supporting improved predictive toxicology. | |
Molecular Weight |
183.16 g/mol |
Source
|
Source | PubChem | |
URL | https://pubchem.ncbi.nlm.nih.gov | |
Description | Data deposited in or computed by PubChem | |
CAS No. |
114425-49-9 |
Source
|
Record name | Benzyl(ethoxy)oxophosphanium | |
Source | EPA DSSTox | |
URL | https://comptox.epa.gov/dashboard/DTXSID20433730 | |
Description | DSSTox provides a high quality public chemistry resource for supporting improved predictive toxicology. | |
体外研究产品的免责声明和信息
请注意,BenchChem 上展示的所有文章和产品信息仅供信息参考。 BenchChem 上可购买的产品专为体外研究设计,这些研究在生物体外进行。体外研究,源自拉丁语 "in glass",涉及在受控实验室环境中使用细胞或组织进行的实验。重要的是要注意,这些产品没有被归类为药物或药品,他们没有得到 FDA 的批准,用于预防、治疗或治愈任何医疗状况、疾病或疾病。我们必须强调,将这些产品以任何形式引入人类或动物的身体都是法律严格禁止的。遵守这些指南对确保研究和实验的法律和道德标准的符合性至关重要。