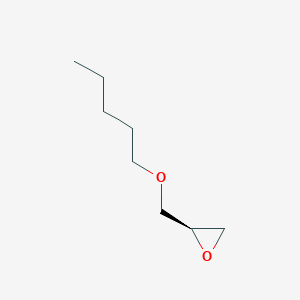
(R)-(+)-Glycidyl pentyl ether
概述
描述
(2R)-2-(pentoxymethyl)oxirane is a chiral epoxide compound characterized by the presence of an oxirane ring and a pentoxymethyl group. Epoxides, also known as oxiranes, are three-membered cyclic ethers that are highly reactive due to the ring strain in their structure. The (2R) configuration indicates the specific stereochemistry of the molecule, which can influence its reactivity and interactions in various chemical processes.
准备方法
Synthetic Routes and Reaction Conditions
The synthesis of (2R)-2-(pentoxymethyl)oxirane typically involves the epoxidation of an appropriate alkene precursor. One common method is the Prilezhaev reaction, where an alkene reacts with a peracid, such as peroxyacetic acid or peroxyformic acid, to form the epoxide . The reaction conditions often include a solvent like dichloromethane and a temperature range of 0-25°C to ensure optimal yield and selectivity.
Industrial Production Methods
Industrial production of (2R)-2-(pentoxymethyl)oxirane may involve continuous flow processes to enhance efficiency and scalability. Flow microreactors, for example, can be used to achieve a more controlled and sustainable synthesis compared to traditional batch processes . These methods allow for precise control over reaction parameters, leading to higher yields and purity of the final product.
化学反应分析
Types of Reactions
(2R)-2-(pentoxymethyl)oxirane undergoes various types of chemical reactions, including:
Oxidation: The epoxide ring can be opened by oxidizing agents to form diols or other oxygenated products.
Reduction: Reductive ring-opening can yield alcohols or other reduced compounds.
Substitution: Nucleophilic substitution reactions can occur at the oxirane ring, leading to the formation of different functionalized products.
Common Reagents and Conditions
Oxidation: Common oxidizing agents include hydrogen peroxide and m-chloroperoxybenzoic acid (mCPBA).
Reduction: Reducing agents like lithium aluminum hydride (LiAlH4) or sodium borohydride (NaBH4) are often used.
Substitution: Nucleophiles such as amines, thiols, and halides can react with the epoxide under basic or acidic conditions.
Major Products
The major products formed from these reactions depend on the specific reagents and conditions used. For example, oxidation with mCPBA can yield diols, while nucleophilic substitution with an amine can produce amino alcohols.
科学研究应用
Polymer Chemistry
Reactive Diluents in Epoxy Resins
- (R)-(+)-Glycidyl pentyl ether is primarily used as a reactive diluent in epoxy formulations. It helps reduce the viscosity of uncured resins, facilitating easier processing and application in adhesives and coatings. This property is crucial for achieving optimal performance in applications such as automotive coatings and electronic encapsulation.
Modification of Biopolymers
- The compound can modify biopolymers like starch and cellulose ethers. By disrupting hydrogen bonding and introducing hydrophobic groups, it enhances the rheological properties of these materials, making them suitable for personal care products such as shampoos and conditioners .
Crosslinking Agents
- In the production of superabsorbent polymers, this compound acts as a crosslinking agent, improving absorbency under load while minimizing gel-blocking effects. This application is particularly relevant in the manufacturing of hygiene products like diapers .
Pharmaceutical Applications
Intermediate in Drug Synthesis
- This compound serves as an intermediate in the synthesis of various pharmaceutical agents. Its reactive nature allows for the incorporation of this compound into larger molecular frameworks necessary for therapeutic efficacy. For instance, it can be utilized in the development of β-blockers and other active pharmaceutical ingredients (APIs) .
Antiviral and Antimalarial Compounds
- Recent studies have indicated that derivatives of this compound can be synthesized to create compounds with antiviral and antimalarial properties, expanding its application in medicinal chemistry .
Chemical Intermediates
Versatile Chemical Linker
- The compound can act as a chemical linker due to its dual functional groups, enabling covalent bonding in various chemical reactions. This property is beneficial for creating complex molecular architectures in organic synthesis .
Scavenger in Chlorinated Solvents
- In solvent systems, this compound functions as an acid scavenger, enhancing stability and performance by neutralizing acidic species that may compromise solvent integrity .
Textile Industry
Surface Modification
- Textiles treated with this compound exhibit improved dye adhesion through permanent chemisorption processes, enhancing color fastness and overall durability of textile products .
Data Table: Applications Overview
Application Area | Specific Use Case | Benefits |
---|---|---|
Polymer Chemistry | Reactive diluent in epoxy resins | Reduced viscosity for easier processing |
Biopolymer Modification | Enhancing properties of starch/cellulose ethers | Improved rheological properties |
Pharmaceutical Synthesis | Intermediate for APIs | Facilitates synthesis of therapeutic agents |
Chemical Intermediates | Scavenger in chlorinated solvents | Enhanced stability |
Textile Industry | Surface modification for improved dye adhesion | Increased color fastness |
Case Studies
-
Epoxy Resin Development
- A study demonstrated that incorporating this compound into epoxy systems significantly improved mechanical properties such as tensile strength and flexibility compared to traditional formulations without this diluent.
-
Pharmaceutical Synthesis
- Research published on the synthesis of a new β-blocker highlighted how this compound was used as an intermediate, showcasing its role in creating compounds with enhanced therapeutic profiles.
-
Textile Treatment Innovations
- A case study on textile dyes indicated that fabrics treated with this compound showed a marked improvement in dye retention under various washing conditions, illustrating its effectiveness as a surface modifier.
作用机制
The mechanism of action of (2R)-2-(pentoxymethyl)oxirane involves the nucleophilic attack on the electrophilic carbon atoms of the oxirane ring. This ring-opening reaction can proceed via different pathways depending on the nature of the nucleophile and the reaction conditions . The molecular targets and pathways involved include interactions with enzymes, proteins, and other biological molecules that can facilitate or inhibit specific biochemical processes.
相似化合物的比较
Similar Compounds
(2R)-Phenoxymethyl-oxirane: Similar in structure but with a phenoxy group instead of a pentoxy group.
(2R)-2-(methoxymethyl)oxirane: Contains a methoxy group instead of a pentoxy group.
(2R)-2-(ethoxymethyl)oxirane: Contains an ethoxy group instead of a pentoxy group.
Uniqueness
(2R)-2-(pentoxymethyl)oxirane is unique due to its specific stereochemistry and the presence of the pentoxymethyl group, which can influence its reactivity and interactions in chemical and biological systems. This uniqueness makes it valuable in applications where specific chiral interactions are crucial, such as in asymmetric synthesis and chiral drug development.
生物活性
(R)-(+)-Glycidyl pentyl ether is an important compound in the field of organic chemistry, particularly known for its applications in the synthesis of various materials and potential biological activities. This article provides a comprehensive overview of the biological activity associated with this compound, including its pharmacological effects, toxicity, and potential therapeutic applications.
Chemical Structure and Properties
This compound is an epoxide compound characterized by a three-membered cyclic ether structure. The presence of the glycidyl group contributes to its reactivity, making it a valuable intermediate in organic synthesis.
1. Toxicological Profile
Research indicates that glycidyl ethers, including this compound, can exhibit significant toxicological effects. The International Agency for Research on Cancer (IARC) has classified certain glycidyl ethers as potential carcinogens based on animal studies that demonstrated tumor formation upon prolonged exposure .
Table 1: Toxicological Data of Glycidyl Ethers
2. Skin Sensitization
Glycidyl ethers are known for their skin sensitization potential. Studies using the Local Lymph Node Assay (LLNA) have shown that these compounds can provoke allergic reactions upon dermal exposure. The sensitization potency varies among different glycidyl ethers, with some exhibiting higher reactivity than others .
Table 2: Skin Sensitization Potency of Selected Glycidyl Ethers
Case Study 1: Immunomodulatory Effects
A study investigated the effects of glycidol derivatives on immune function in murine models. Results indicated that these compounds could enhance antibody production and modulate cytokine profiles, suggesting potential applications in immunotherapy .
Case Study 2: Carcinogenicity Assessment
In a long-term inhalation study involving phenyl glycidyl ether, significant incidences of nasal tumors were reported in rats exposed to high concentrations. This study highlights the importance of understanding the carcinogenic potential of glycidyl ethers and emphasizes the need for careful handling and assessment of these compounds in industrial settings .
属性
IUPAC Name |
(2R)-2-(pentoxymethyl)oxirane | |
---|---|---|
Source | PubChem | |
URL | https://pubchem.ncbi.nlm.nih.gov | |
Description | Data deposited in or computed by PubChem | |
InChI |
InChI=1S/C8H16O2/c1-2-3-4-5-9-6-8-7-10-8/h8H,2-7H2,1H3/t8-/m0/s1 | |
Source | PubChem | |
URL | https://pubchem.ncbi.nlm.nih.gov | |
Description | Data deposited in or computed by PubChem | |
InChI Key |
IQCASZIDTNHBIW-QMMMGPOBSA-N | |
Source | PubChem | |
URL | https://pubchem.ncbi.nlm.nih.gov | |
Description | Data deposited in or computed by PubChem | |
Canonical SMILES |
CCCCCOCC1CO1 | |
Source | PubChem | |
URL | https://pubchem.ncbi.nlm.nih.gov | |
Description | Data deposited in or computed by PubChem | |
Isomeric SMILES |
CCCCCOC[C@H]1CO1 | |
Source | PubChem | |
URL | https://pubchem.ncbi.nlm.nih.gov | |
Description | Data deposited in or computed by PubChem | |
Molecular Formula |
C8H16O2 | |
Source | PubChem | |
URL | https://pubchem.ncbi.nlm.nih.gov | |
Description | Data deposited in or computed by PubChem | |
Molecular Weight |
144.21 g/mol | |
Source | PubChem | |
URL | https://pubchem.ncbi.nlm.nih.gov | |
Description | Data deposited in or computed by PubChem | |
Synthesis routes and methods I
Procedure details
Synthesis routes and methods II
Procedure details
体外研究产品的免责声明和信息
请注意,BenchChem 上展示的所有文章和产品信息仅供信息参考。 BenchChem 上可购买的产品专为体外研究设计,这些研究在生物体外进行。体外研究,源自拉丁语 "in glass",涉及在受控实验室环境中使用细胞或组织进行的实验。重要的是要注意,这些产品没有被归类为药物或药品,他们没有得到 FDA 的批准,用于预防、治疗或治愈任何医疗状况、疾病或疾病。我们必须强调,将这些产品以任何形式引入人类或动物的身体都是法律严格禁止的。遵守这些指南对确保研究和实验的法律和道德标准的符合性至关重要。