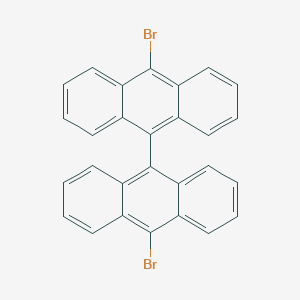
10,10'-Dibromo-9,9'-bianthracene
描述
10,10′-Dibromo-9,9′-bianthracene (DBBA, C₂₈H₁₆Br₂) is a halogenated bianthracene derivative widely used in the bottom-up synthesis of atomically precise graphene nanoribbons (GNRs). Its synthesis involves anthraquinone as a starting material, bromination with N-bromosuccinimide (NBS), and reduction with zinc and hydrochloric acid, achieving a yield of 72.3% . DBBA’s utility stems from its two bromine atoms at the 10,10′ positions, which undergo selective dehalogenation on catalytic metal surfaces (e.g., Au, Ag, Cu) to form biradicals. These intermediates polymerize via Ullmann coupling and cyclodehydrogenation (200–400°C) to yield armchair GNRs (7-AGNRs) with widths of ~0.7 nm .
DBBA’s on-surface reactivity is substrate-dependent. On Au(111), it forms linear polymers that planarize into 7-AGNRs, while on Cu(111), dehydrogenative coupling at 2,2′ positions produces chiral zigzag GNRs. Ag(111) enables simultaneous dehalogenation and cyclodehydrogenation, forming armchair GNRs through graphene platelet fusion . Substrate interactions, reaction kinetics, and monomer design critically influence DBBA’s structural outcomes .
准备方法
10,10’-Dibromo-9,9’-bianthracene can be synthesized through the bromination of 9,9’-bianthracene. This reaction typically occurs in the presence of a brominating agent such as bromine (Br2) in a solvent like chloromethane . The reaction conditions need to be carefully controlled to ensure the selective bromination at the 10 and 10’ positions.
化学反应分析
10,10’-Dibromo-9,9’-bianthracene undergoes several types of chemical reactions, including:
Dehalogenation: This reaction involves the removal of bromine atoms, often facilitated by a reducing agent.
Debromination: Similar to dehalogenation, debromination specifically targets the removal of bromine atoms.
Ullmann Coupling: This reaction involves the coupling of aryl halides in the presence of a copper catalyst, leading to the formation of biaryl compounds.
Common reagents used in these reactions include reducing agents for dehalogenation and copper catalysts for Ullmann coupling. The major products formed from these reactions depend on the specific conditions and reagents used.
科学研究应用
Organic Electronics
Role as a Precursor:
10,10'-Dibromo-9,9'-bianthracene serves as a crucial precursor in the development of organic light-emitting diodes (OLEDs) and organic photovoltaics (OPVs). Its unique molecular structure allows for effective electronic interactions, making it suitable for creating materials that exhibit specific light-emitting or light-harvesting properties.
Synthesis of Graphene Nanoribbons:
The compound has been extensively studied for its ability to facilitate the bottom-up synthesis of graphene nanoribbons (GNRs). Research indicates that this compound can be used to produce armchair graphene nanoribbons through thermal activation on metal surfaces such as silver and gold. The bromine atoms play a vital role in controlling the polymerization process during GNR synthesis .
Material Science
Nanostructure Formation:
The compound's ability to form well-ordered monolayers on crystalline surfaces is essential for the fabrication of long and oriented GNRs. Studies have shown that when deposited on surfaces like Au(111), it self-assembles into a dense monolayer that enhances the efficiency of polymerization processes, leading to more controlled growth of GNRs compared to conventional methods .
Chemical Interactions:
Research has demonstrated that this compound undergoes significant changes upon adsorption on metal surfaces. For instance, it can partially debrominate at room temperature and completely lose bromine atoms at elevated temperatures. These transformations are critical for understanding its reactivity and potential applications in material synthesis .
Case Studies
作用机制
The mechanism of action of 10,10’-Dibromo-9,9’-bianthracene in the synthesis of graphene nanoribbons involves several steps:
Self-Assembly: The compound self-assembles into a well-ordered dense monolayer on metal surfaces such as gold (Au) at room temperature.
Formation of GNRs: The polymerized structure results in the formation of long and oriented graphene nanoribbons, which are essential for high-performance electronic devices.
相似化合物的比较
Structural Analogs with Modified Halogen Positions
2,2′-Dibromo-9,9′-bianthracene
- Structure : Bromine substituents at 2,2′ positions instead of 10,10′.
- Reactivity : On Cu(111), both DBBA and 2,2′-dibromo-9,9′-bianthracene form chiral (3,1)-GNRs. However, 2,2′-substitution minimizes substrate interactions, ensuring Ullmann coupling proceeds uniformly across Au(111), Ag(111), and Cu(111) .
- Outcome : Chiral GNRs with distinct edge geometries compared to DBBA-derived 7-AGNRs.
2,2′-Diphenyl-10,10′-dibromo-9,9′-bianthracene (DP-DBBA)
- Structure : Adds phenyl groups at 2,2′ positions to DBBA’s anthracene branches.
- Reactivity: The phenyl groups sterically hinder surface interactions, altering polymerization pathways. On Au(111), DP-DBBA forms coordination polymers with chiral imprinting, whereas DBBA yields linear 7-AGNRs. On Ag(111), DP-DBBA generates nanoporous graphene instead of fused armchair GNRs .
- Outcome: Diverse products (coordination polymers, nanoporous graphene) due to suppressed cyclodehydrogenation and enhanced monomer rigidity.
Functional Group Modifications
10,10′-Distyryl-9,9′-bianthracene (DSBA)
- Structure : Styryl groups replace bromines via Heck coupling with DBBA .
- Reactivity: Enhanced solubility and altered conjugation length.
Dimethyl- and Diamine-Substituted Bianthracenes
- Structure : 10,10′-dimethyl (Me₂BA) and 10,10′-diamine ((NH₂)₂BA) derivatives.
- Reactivity : Reduced directionality in self-assembly on Cu(111) compared to DBBA. Me₂BA and (NH₂)₂BA form disordered or amorphous structures, whereas DBBA’s bromines drive ordered chain-like assemblies .
Substrate-Dependent Reactivity of DBBA and Analogs
Compound | Substrate | Reaction Pathway | Product | Key Difference vs. DBBA |
---|---|---|---|---|
DBBA | Au(111) | Ullmann coupling → cyclodehydrogenation | 7-AGNRs | Baseline for armchair GNR synthesis |
DBBA | Cu(111) | Dehydrogenative coupling at 2,2′ sites | Chiral zigzag GNRs | Altered edge geometry |
DBBA | Ag(111) | Simultaneous dehalogenation + planarization | Armchair GNRs via platelets | Unique intermediate fusion pathway |
2,2′-dibromo analog | Cu(111) | Ullmann coupling at 2,2′ sites | Chiral (3,1)-GNRs | Uniform reactivity across substrates |
DP-DBBA | Au(111) | Coordination polymerization | Chiral polymer chains | No GNR formation due to steric effects |
Kinetic and Thermodynamic Considerations
- DBBA vs. 2,2′-Dibromo Analog : DBBA’s 10,10′-bromines favor kinetically controlled Ullmann coupling on Au(111), while 2,2′-substitution thermodynamically stabilizes chiral edge formation on Cu(111) .
- Role of Organometallic Intermediates: DBBA on Ag(111) forms organometallic (OM) chains that polymerize into polyanthracene, whereas on Cu(110), strong substrate bonding arrests polymerization, yielding nanographene-Br adducts .
Key Research Findings
Substrate Catalysis : Ni adatoms on Au(111) enhance DBBA’s cyclodehydrogenation, producing wider fused GNRs (14–28 carbon widths) .
Self-Assembly Control: DBBA’s ordered monolayers on Au(111) enable aligned GNR growth, achieving longer ribbons than conventional methods .
Competing Reactions : DBBA’s dehalogenation competes with metalation/hydrogenation, requiring precise control to favor Ullmann coupling .
生物活性
10,10'-Dibromo-9,9'-bianthracene (DBBA) is a halogenated bianthracene compound that has garnered interest in various fields, particularly in organic electronics and materials science. Its unique structural properties facilitate its use in the synthesis of advanced materials such as graphene nanoribbons. However, its biological activity is an area of emerging research that warrants detailed exploration.
DBBA is characterized by the presence of bromine atoms at the 10 positions of the bianthracene structure. This substitution significantly affects its reactivity and interactions with biological systems. The compound's ability to undergo debromination under specific conditions also plays a crucial role in its biological effects.
The biological activity of DBBA is believed to stem from its interactions with cellular components. The bromine atoms enhance the electrophilicity of the compound, allowing it to participate in electron transfer reactions. This reactivity can influence various cellular processes, including:
- Signal Transduction : DBBA may alter signaling pathways by interacting with proteins involved in these processes.
- Gene Expression : The compound could modulate gene expression by affecting transcription factors or other regulatory proteins.
Research indicates that DBBA can bind to specific biomolecules, leading to alterations in their activity and subsequent biological effects .
Biological Studies and Case Reports
Recent studies have begun to investigate the potential therapeutic applications of DBBA. For instance:
- Anticancer Activity : Preliminary studies suggest that DBBA derivatives exhibit cytotoxic effects on cancer cell lines. The mechanism may involve the generation of reactive oxygen species (ROS) that induce apoptosis in malignant cells .
- Antimicrobial Properties : Some derivatives of DBBA have shown potential antimicrobial activity, possibly due to their ability to disrupt bacterial cell membranes or interfere with metabolic processes .
Table 1: Summary of Biological Activities of DBBA Derivatives
Activity Type | Effect | Mechanism |
---|---|---|
Anticancer | Cytotoxicity to cancer cells | Induction of apoptosis via ROS generation |
Antimicrobial | Inhibition of bacterial growth | Disruption of cell membranes |
Cell Signaling | Modulation of signaling pathways | Interaction with proteins involved in signaling |
Synthesis and Applications
DBBA is primarily synthesized through bromination reactions involving bianthracene. This synthesis allows for the production of various derivatives that may enhance its biological activity. For example, modifications can be made to improve solubility or target specific cellular pathways.
In materials science, DBBA is utilized as a precursor for the bottom-up synthesis of graphene nanoribbons (GNRs). The self-assembly properties on metal surfaces such as gold (Au) and silver (Ag) have been extensively studied, revealing insights into how these structures can be controlled for electronic applications .
Research Findings
Recent investigations have highlighted the following key findings regarding DBBA:
- Self-Assembly on Metal Surfaces : Scanning tunneling microscopy studies show that DBBA forms well-ordered monolayers on Au(111) and Ag(111), which are crucial for GNR synthesis .
- Debromination Effects : At elevated temperatures, DBBA undergoes debromination, leading to different reaction pathways that can influence both material properties and biological interactions .
- Potential Drug Candidate : Ongoing research is exploring the viability of DBBA as a drug candidate due to its unique properties and mechanisms of action against cancer cells .
常见问题
Basic Research Questions
Q. What experimental protocols are recommended for synthesizing graphene nanoribbons (GNRs) using DBBA?
DBBA is a precursor for 7-atom-wide armchair GNRs (7-AGNRs) via on-surface synthesis. Key steps include:
- Deposition : Sublimate DBBA onto metal substrates (e.g., Au(111), Cu(111)) under ultrahigh vacuum (UHV) to ensure monolayer formation .
- Thermal Activation : Anneal at 200–300°C to trigger dehalogenation and Ullmann coupling, forming polymeric chains. Subsequent cyclodehydrogenation at 400–450°C yields planar GNRs .
- Substrate Choice : Au(111) favors straight 7-AGNRs via Ullmann coupling, while Cu(111) may induce chiral edge structures through dehydrogenative coupling .
Q. How does substrate selection influence DBBA polymerization and GNR morphology?
Substrate catalytic activity dictates reaction pathways:
- Au(111) : Promotes Ullmann coupling (C–Br cleavage) for armchair GNRs .
- Cu(111) : Enables dehydrogenative coupling, forming chiral zigzag edges due to stronger surface interactions .
- Ag(111) : Induces simultaneous dehalogenation and cyclodehydrogenation, bypassing polymerization and forming graphene platelets .
STM and XPS are critical for verifying intermediate structures and reaction completeness .
Q. What deposition methods mitigate DBBA degradation during sublimation?
High molecular weight and thermal sensitivity necessitate alternative deposition strategies:
- Direct Contact Transfer (DCT) : Use a pre-coated stamp to transfer DBBA onto substrates without sublimation, preserving molecular integrity .
- Low-Temperature Sublimation : Optimize source temperature (e.g., 160°C) and UHV conditions to minimize decomposition .
Q. Which characterization techniques validate DBBA-derived GNRs?
- STM : Resolves atomic-scale ribbon width, edge chirality, and defects .
- XPS/UPS : Tracks dehalogenation (Br 3d peak attenuation) and electronic structure evolution .
- Raman Spectroscopy : Confirms GNR quality via D/G band intensity ratios, even for substrates like nickel foil .
Advanced Research Questions
Q. How do reaction kinetics govern DBBA’s coupling pathways on different substrates?
Kinetic control arises from:
- Halogen Position : 10,10′-dibromination favors Ullmann coupling on Au(111), while 2,2′-dibromination directs chiral edge formation on Cu(111) .
- Temperature Gradients : Stepwise annealing (200°C → 450°C) separates dehalogenation, polymerization, and cyclodehydrogenation, preventing side reactions .
- Coverage Density : High precursor density on Au(111) aligns monomers for linear polymerization, whereas low density on Cu(111) permits rotational freedom and chiral coupling .
Q. Can DBBA be integrated into heterojunctions for tailored electronic properties?
Yes, by co-depositing DBBA with structurally distinct monomers (e.g., 2,2’-di-biphenyl-DBBA):
- Width Modulation : Combine DBBA (N=7 GNRs) with wider precursors (N=13 GNRs) to create heterojunctions with quantum confinement effects .
- Electronic Decoupling : Use Cu3Au(111) substrates to reduce itinerant electron interference, enabling spin-polarized measurements .
Q. What strategies control edge structure in DBBA-derived GNRs?
- Substrate Engineering : Au(110) templates straight edges, while Cu(111) induces kinked or chiral configurations .
- Monomer Functionalization : Introduce phenyl groups (e.g., DP-DBBA) to sterically hinder undesired coupling pathways, enforcing edge-extended zigzag motifs .
Q. How does DBBA’s environmental hazard profile impact laboratory handling?
属性
IUPAC Name |
9-bromo-10-(10-bromoanthracen-9-yl)anthracene | |
---|---|---|
Source | PubChem | |
URL | https://pubchem.ncbi.nlm.nih.gov | |
Description | Data deposited in or computed by PubChem | |
InChI |
InChI=1S/C28H16Br2/c29-27-21-13-5-1-9-17(21)25(18-10-2-6-14-22(18)27)26-19-11-3-7-15-23(19)28(30)24-16-8-4-12-20(24)26/h1-16H | |
Source | PubChem | |
URL | https://pubchem.ncbi.nlm.nih.gov | |
Description | Data deposited in or computed by PubChem | |
InChI Key |
NPNNLGXEAGTSRN-UHFFFAOYSA-N | |
Source | PubChem | |
URL | https://pubchem.ncbi.nlm.nih.gov | |
Description | Data deposited in or computed by PubChem | |
Canonical SMILES |
C1=CC=C2C(=C1)C(=C3C=CC=CC3=C2Br)C4=C5C=CC=CC5=C(C6=CC=CC=C64)Br | |
Source | PubChem | |
URL | https://pubchem.ncbi.nlm.nih.gov | |
Description | Data deposited in or computed by PubChem | |
Molecular Formula |
C28H16Br2 | |
Source | PubChem | |
URL | https://pubchem.ncbi.nlm.nih.gov | |
Description | Data deposited in or computed by PubChem | |
DSSTOX Substance ID |
DTXSID70569912 | |
Record name | 10,10'-Dibromo-9,9'-bianthracene | |
Source | EPA DSSTox | |
URL | https://comptox.epa.gov/dashboard/DTXSID70569912 | |
Description | DSSTox provides a high quality public chemistry resource for supporting improved predictive toxicology. | |
Molecular Weight |
512.2 g/mol | |
Source | PubChem | |
URL | https://pubchem.ncbi.nlm.nih.gov | |
Description | Data deposited in or computed by PubChem | |
CAS No. |
121848-75-7 | |
Record name | 10,10'-Dibromo-9,9'-bianthracene | |
Source | EPA DSSTox | |
URL | https://comptox.epa.gov/dashboard/DTXSID70569912 | |
Description | DSSTox provides a high quality public chemistry resource for supporting improved predictive toxicology. | |
Retrosynthesis Analysis
AI-Powered Synthesis Planning: Our tool employs the Template_relevance Pistachio, Template_relevance Bkms_metabolic, Template_relevance Pistachio_ringbreaker, Template_relevance Reaxys, Template_relevance Reaxys_biocatalysis model, leveraging a vast database of chemical reactions to predict feasible synthetic routes.
One-Step Synthesis Focus: Specifically designed for one-step synthesis, it provides concise and direct routes for your target compounds, streamlining the synthesis process.
Accurate Predictions: Utilizing the extensive PISTACHIO, BKMS_METABOLIC, PISTACHIO_RINGBREAKER, REAXYS, REAXYS_BIOCATALYSIS database, our tool offers high-accuracy predictions, reflecting the latest in chemical research and data.
Strategy Settings
Precursor scoring | Relevance Heuristic |
---|---|
Min. plausibility | 0.01 |
Model | Template_relevance |
Template Set | Pistachio/Bkms_metabolic/Pistachio_ringbreaker/Reaxys/Reaxys_biocatalysis |
Top-N result to add to graph | 6 |
Feasible Synthetic Routes
体外研究产品的免责声明和信息
请注意,BenchChem 上展示的所有文章和产品信息仅供信息参考。 BenchChem 上可购买的产品专为体外研究设计,这些研究在生物体外进行。体外研究,源自拉丁语 "in glass",涉及在受控实验室环境中使用细胞或组织进行的实验。重要的是要注意,这些产品没有被归类为药物或药品,他们没有得到 FDA 的批准,用于预防、治疗或治愈任何医疗状况、疾病或疾病。我们必须强调,将这些产品以任何形式引入人类或动物的身体都是法律严格禁止的。遵守这些指南对确保研究和实验的法律和道德标准的符合性至关重要。