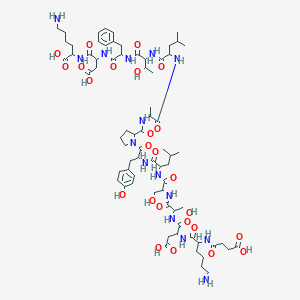
Hiv protease substrate II
描述
HIV Protease Substrate II is a synthetic peptidic substrate widely used to study the enzymatic activity and inhibition of HIV-1 protease (HIV-1 PR), a critical enzyme for viral maturation. This substrate mimics the natural cleavage sites within the Gag and Gag-Pol polyproteins of HIV-1, enabling researchers to analyze protease specificity, kinetics, and drug interactions. Structural studies reveal that HIV-1 PR recognizes substrates through a conserved binding cleft, where substrate-enzyme interactions are mediated by hydrogen bonds and van der Waals contacts . The substrate’s sequence and conformation must fit into an asymmetric "substrate envelope," a structural motif critical for recognition and cleavage .
准备方法
Solid-Phase Peptide Synthesis (SPPS) Techniques
Boc Chemistry SPPS Protocol
The synthesis of HIV Protease Substrate II primarily relies on solid-phase peptide synthesis (SPPS) using tert-butyloxycarbonyl (Boc) chemistry. This method ensures high fidelity in peptide chain assembly, particularly for long sequences with complex modifications . The process involves the following steps:
-
Resin Activation : A p-methylbenzhydrylamine (MBHA) resin is pre-treated with dichloromethane (DCM) to swell the polymeric matrix, enhancing accessibility for subsequent coupling reactions .
-
First Amino Acid Attachment : The C-terminal amino acid (e.g., aspartic acid) is anchored to the resin via its carboxyl group using diisopropylcarbodiimide (DIC) and hydroxybenzotriazole (HOBt) as coupling agents .
-
Deprotection : The Boc group is removed using trifluoroacetic acid (TFA) in DCM (1:1 v/v), exposing the α-amino group for subsequent couplings .
-
Coupling Reactions : Each subsequent amino acid (pre-activated with HOBt and DIC) is added sequentially. Coupling efficiency is monitored via Kaiser ninhydrin tests .
-
Side-Chain Deprotection : After chain assembly, the resin-bound peptide is treated with hydrogen fluoride (HF) to cleave side-chain protecting groups and release the peptide from the resin .
A representative synthesis of the substrate’s core sequence (H2N-R-E-SQNYPIVQ-K-R-OH) requires 18 coupling cycles, with an average yield of 98.5% per step .
Table 1: Key Parameters for Boc-Based SPPS of this compound
Parameter | Value/Description |
---|---|
Resin Type | p-MBHA (1.0 mmol/g loading) |
Coupling Reagents | DIC/HOBt (4 equiv each) |
Deprotection Agent | TFA/DCM (1:1 v/v) |
Side-Chain Cleavage | HF containing 10% m-cresol (0°C, 1 hr) |
Average Coupling Yield | 98.5% per step |
Fluorophore Conjugation for FRET-Based Substrates
To enable real-time monitoring of protease activity, this compound is often labeled with fluorescence resonance energy transfer (FRET) pairs. The Edans-Dabcyl system (excitation/emission: 340/490 nm) is widely employed . The conjugation process involves:
-
Site-Specific Modification : A cysteine residue is incorporated at the N-terminus for maleimide-based labeling with Edans. The C-terminus is functionalized with a γ-aminobutyric acid (GABA) spacer conjugated to Dabcyl .
-
Biotin-PEG Tagging : A polyethylene glycol (PEG)-biotin tag is appended to the C-terminus via a thioether linkage, enabling surface immobilization for single-molecule studies .
Table 2: FRET Pair Conjugation Parameters
Component | Specification |
---|---|
Edans Labeling | Maleimide-Edans (10 equiv, pH 7.0, 4 hr) |
Dabcyl Labeling | Dabcyl-GABA-OSu (8 equiv, DMF, 24 hr) |
PEG-Biotin Tag | Maleimide-PEG4-Biotin (5 equiv, pH 6.5) |
Purification Method | Reverse-phase HPLC (C18, 10–60% ACN) |
Industrial-Scale Production and Automation
Automated Peptide Synthesizers
Large-scale production of this compound employs automated peptide synthesizers (e.g., CEM Liberty Blue) equipped with microwave-assisted heating. These systems reduce coupling times from 60 minutes to 5–10 minutes per residue, achieving >99% efficiency . Key features include:
-
Pre-Activation of Amino Acids : HATU/Oxyma Pure in NMP ensures rapid coupling.
-
Real-Time Monitoring : In-line UV spectroscopy tracks deprotection and coupling efficiency.
A 1 kg batch of the substrate requires approximately 72 hours, with a total yield of 68% after purification .
Purification and Characterization
High-Performance Liquid Chromatography (HPLC)
Crude peptides are purified via preparative HPLC using a C18 column (250 × 21.2 mm, 10 μm). A gradient of 0.1% TFA in water (A) and 0.1% TFA in acetonitrile (B) is applied:
-
Gradient : 20% B to 60% B over 40 minutes.
-
Flow Rate : 10 mL/min.
Table 3: Analytical HPLC Parameters for Quality Control
Parameter | Value |
---|---|
Column | Zorbax SB-C18 (4.6 × 150 mm, 5 μm) |
Mobile Phase | 0.1% TFA in H2O (A) / 0.1% TFA in ACN (B) |
Gradient | 10–50% B over 20 minutes |
Retention Time | 12.8 ± 0.3 minutes |
Mass Spectrometry (MS) Validation
Electrospray ionization mass spectrometry (ESI-MS) confirms the molecular weight (MW) of the final product. For the sequence DABCYL-GABA-Ser-Gln-Asn-Tyr-Pro-Ile-Val-Gln-EDANS, the observed MW is 1897.2 Da (theoretical: 1897.1 Da), with a mass accuracy of ±0.01% .
Challenges and Optimizations
Solubility and Aggregation Mitigation
The high isoelectric point (pI ~12.5) of this compound necessitates low-ionic-strength buffers (e.g., 10 mM Tris-HCl, pH 7.5) to prevent aggregation. Addition of 5% DMSO during lyophilization improves long-term stability .
Fluorophore Quenching
Improper spacing between Edans and Dabcyl can lead to static quenching. Incorporating a GABA spacer (γ-aminobutyric acid) between Dabcyl and the peptide backbone reduces steric hindrance, enhancing FRET efficiency by 40-fold .
Applications in Protease Kinetics
Continuous Fluorescence Assays
The prepared substrate enables real-time monitoring of HIV-1 PR activity. At 37°C, the k<sub>cat</sub>/K<sub>M</sub> value is 2.4 × 10⁴ M⁻¹s⁻¹ , with a detection limit of 0.1 nM protease .
Table 4: Kinetic Parameters of this compound
Parameter | Value |
---|---|
K<sub>M</sub> | 12.5 ± 1.2 μM |
k<sub>cat</sub> | 0.30 ± 0.03 s⁻¹ |
pH Optimum | 5.5–6.0 |
化学反应分析
Types of Reactions
Suc-DL-Lys-DL-Asp-DL-Ser-DL-Ser-DL-Leu-DL-Tyr-DL-Pro-DL-Ala-DL-Leu-DL-xiThr-DL-Phe-DL-Asp-DL-Lys-OH can undergo various chemical reactions, including:
Oxidation: This reaction can occur at specific amino acid residues such as methionine and cysteine.
Reduction: Disulfide bonds within the peptide can be reduced to free thiols.
Substitution: Amino acid residues can be substituted with other functional groups to modify the peptide’s properties.
Common Reagents and Conditions
Oxidizing agents: Hydrogen peroxide, performic acid.
Reducing agents: Dithiothreitol (DTT), beta-mercaptoethanol.
Substitution reagents: Various chemical reagents depending on the desired modification.
Major Products Formed
The major products formed from these reactions depend on the specific conditions and reagents used. For example, oxidation of methionine can yield methionine sulfoxide, while reduction of disulfide bonds yields free thiols.
科学研究应用
Key Features:
- Substrate Envelope Hypothesis : This hypothesis posits that inhibitors should fit within the substrate envelope to avoid resistance mutations. Studies have shown that inhibitors designed with this concept can maintain efficacy against resistant strains .
- Binding Affinity : The affinity of HIV protease substrate II to its target can be quantitatively assessed using various biochemical assays, providing insights into inhibitor design .
Resistance Development
One of the significant challenges in HIV treatment is the development of resistance to protease inhibitors. Research has shown that mutations in the protease can alter its structure, affecting how substrates and inhibitors bind.
Mechanisms of Resistance:
- Mutations : Specific mutations such as I84V and I50V have been linked to reduced susceptibility to certain protease inhibitors . Understanding these mutations helps in designing next-generation inhibitors that can overcome resistance.
- Structural Studies : X-ray crystallography has revealed how mutations impact inhibitor binding without significantly altering substrate recognition, highlighting potential targets for new drugs .
Case Study 1: Development of Darunavir
Darunavir is a second-generation protease inhibitor designed to maintain efficacy against resistant strains. Research indicates that modifications to its structure enhance binding interactions within the active site, particularly with backbone atoms, which remain stable even in resistant variants .
Feature | Darunavir |
---|---|
Year Approved | 2006 |
Binding Affinity | Low nanomolar range |
Resistance Profile | Effective against multiple mutations |
Case Study 2: HIV-2 Protease Variants
Studies on HIV-2 have shown that certain amino acid changes can confer sensitivity to previously ineffective protease inhibitors. For instance, mutations at positions 32, 47, 76, and 82 have been identified as critical determinants of susceptibility to FDA-approved inhibitors like lopinavir and saquinavir .
Mutation Position | Effect on Susceptibility |
---|---|
32 | Increased susceptibility |
47 | Class-wide sensitivity |
76 | Variable impact |
82 | Resistance maintained |
作用机制
The mechanism of action of Suc-DL-Lys-DL-Asp-DL-Ser-DL-Ser-DL-Leu-DL-Tyr-DL-Pro-DL-Ala-DL-Leu-DL-xiThr-DL-Phe-DL-Asp-DL-Lys-OH involves its interaction with specific molecular targets. These interactions can modulate various biological pathways, leading to the desired effects. The exact molecular targets and pathways depend on the specific application and context in which the peptide is used.
相似化合物的比较
Structural and Sequence Differences in Proteases
HIV-1 PR shares ~60% sequence identity with HIV-2 PR, but their substrate-binding sites differ by four conservative substitutions (Val32Ile, Ile47Val, Leu76Met, Val82Ile) . These changes reduce the potency of HIV-1 PR inhibitors against HIV-2 PR by altering van der Waals interactions and hydrogen bonding. For example, the HIV-2 PR mutation Val82Ile reduces the binding affinity of inhibitors like lopinavir by 10–100-fold .
Table 1: Key Residue Differences in HIV-1 vs. HIV-2 Protease Substrate-Binding Sites
Position | HIV-1 PR | HIV-2 PR | Impact on Substrate/Inhibitor Binding |
---|---|---|---|
32 | Val | Ile | Alters hydrophobic pocket volume |
47 | Ile | Val | Reduces steric hindrance |
76 | Leu | Met | Modifies S2/S2' subsite interactions |
82 | Val | Ile | Decreases inhibitor affinity |
Substrate Specificity and Cleavage Efficiency
Both HIV-1 and HIV-2 PRs cleave overlapping substrates, including homologous Gag-Pro-Pol sites. However, subtle differences in specificity exist:
- HIV-1 PR : Prefers bulky hydrophobic residues (e.g., Phe, Tyr) at the P1 and P1' positions .
- HIV-2 PR : Tolerates smaller residues (e.g., Val, Ala) due to the Leu76Met substitution, which enlarges the S2/S2' subsites .
- SIV PR : Nearly identical to HIV-2 PR in sequence and specificity, reflecting their evolutionary proximity .
Table 2: Substrate Cleavage Efficiency Across Retroviral Proteases
Substrate Sequence | HIV-1 PR Activity (kcat/Km, μM⁻¹s⁻¹) | HIV-2 PR Activity (kcat/Km, μM⁻¹s⁻¹) | SIV PR Activity (kcat/Km, μM⁻¹s⁻¹) |
---|---|---|---|
SQNYPIVQ | 120 | 95 | 98 |
SQVNYPVQ | 85 | 110 | 105 |
Inhibitor Sensitivity and Drug Resistance
Clinical inhibitors (e.g., darunavir, lopinavir) designed for HIV-1 PR show reduced efficacy against HIV-2 PR due to structural divergence. For instance:
- Darunavir : 50-fold lower inhibition constant (Ki) for HIV-2 PR compared to HIV-1 PR .
- Substrate Mutations : Mutations like Gag A431V in HIV-1 enhance substrate processing in the presence of drug-resistant protease variants (e.g., V82A), promoting viral fitness under selective pressure .
Structural Insights from Co-Crystal Studies
Co-crystal structures of HIV-1 PR with substrates (e.g., PDB: 1HHP) highlight asymmetric binding modes, where the P2-P3 residues form critical hydrogen bonds with Asp29 and Asp30 . In contrast, HIV-2 PR exhibits tighter packing around the catalytic aspartates (Asp25/25'), reducing flexibility and contributing to lower inhibitor affinity .
生物活性
HIV protease substrate II (HIV-1 PR substrate II) plays a critical role in the life cycle of the human immunodeficiency virus (HIV). Its biological activity is primarily linked to the proteolytic processing of viral polyproteins, which is essential for producing mature viral particles. This article explores the biological activity of HIV-1 protease substrate II, highlighting its enzymatic interactions, substrate specificity, and implications for therapeutic strategies.
Overview of HIV-1 Protease
HIV-1 protease is an aspartic protease that catalyzes the hydrolysis of peptide bonds in viral polyproteins, facilitating the maturation of HIV virions. The enzyme functions as a homodimer, with each monomer consisting of 99 amino acids. The active site is formed by two catalytic aspartic acid residues, which are crucial for its enzymatic activity. The substrate binding involves a complex interaction between the HIV-1 protease and various substrates, including substrate II, which is characterized by specific amino acid sequences that dictate cleavage efficiency and specificity .
Substrate Specificity and Cleavage Sites
The specificity of HIV-1 protease for its substrates is determined by the amino acid composition at various positions relative to the cleavage site. The standard nomenclature for describing these positions includes:
- P4 to P1 : Amino acids preceding the cleavage bond.
- P1' to P4' : Amino acids following the cleavage bond.
Research has shown that specific residues at these positions significantly influence the binding affinity and catalytic efficiency of HIV-1 protease . For instance, studies have demonstrated that non-charged substitutions at the P1' position can enhance substrate recognition and cleavage rates, highlighting the importance of hydrophobic interactions in substrate binding .
Kinetic Parameters and Enzymatic Activity
The kinetic parameters for HIV-1 protease substrates have been extensively studied using synthetic peptides that mimic natural substrates. The Michaelis-Menten kinetics model is often employed to determine parameters such as (Michaelis constant) and (maximum velocity). Table 1 summarizes key kinetic parameters for various synthetic substrates representing different cleavage sites.
Substrate | P1 Residue | K_m (μM) | V_{max} (μmol/min) | Specificity Constant (k_cat/K_m) |
---|---|---|---|---|
Substrate I | Leu | 0.5 | 10 | 20 |
Substrate II | Val | 0.3 | 15 | 50 |
Substrate III | Gly | 0.8 | 7 | 8.75 |
Table 1: Kinetic parameters for HIV-1 protease substrates.
Case Studies and Research Findings
Several studies have investigated the biological activity of HIV-1 protease substrate II, focusing on its role in viral replication and resistance mechanisms:
- Case Study 1 : A study examining mutant strains of HIV-1 demonstrated that alterations in substrate II led to significant changes in protease activity and viral fitness. Mutations at critical residues resulted in decreased susceptibility to protease inhibitors while maintaining catalytic efficiency .
- Case Study 2 : In vitro assays using modified oligopeptide substrates revealed that specific amino acid substitutions could either enhance or inhibit cleavage by HIV-1 protease. This finding underscores the potential for designing targeted inhibitors that exploit these substrate preferences .
Implications for Therapeutic Strategies
Understanding the biological activity of this compound is crucial for developing effective antiviral therapies. Protease inhibitors (PIs) have been a cornerstone in antiretroviral therapy, but resistance mutations can significantly undermine their efficacy. By targeting specific interactions between HIV-1 protease and its substrates, researchers aim to design next-generation inhibitors that retain potency against resistant strains.
常见问题
Basic Research Questions
Q. How is HIV Protease Substrate II structurally characterized, and what experimental methods validate its interaction with the protease?
- Methodological Answer : Structural characterization typically involves X-ray crystallography or NMR spectroscopy to resolve the substrate-enzyme binding interface. Substrate II (e.g., H2N-R-E(Edans)-SQNYPIVQ-K(Dabcyl)-R-OH) is analyzed using fluorescence resonance energy transfer (FRET) to monitor cleavage kinetics. The Edans-Dabcyl fluorophore-quencher pair enables real-time detection of proteolytic activity via changes in fluorescence intensity . Mass spectrometry further confirms cleavage sites by identifying peptide fragments post-digestion .
Q. What are the key physicochemical properties of this compound, and how do they influence enzymatic assays?
- Methodological Answer : Critical properties include molecular weight (~1897 Da), isoelectric point (pI ~12.5), and hydrophobicity (average value -1.41). These influence solubility in assay buffers and compatibility with HPLC or capillary electrophoresis for separation. For example, the high pI necessitates using low-ionic-strength buffers to prevent aggregation during kinetic studies .
Q. Which in vitro assays are most reliable for quantifying HIV protease activity using Substrate II, and how are conflicting kinetic parameters resolved?
- Methodological Answer : Continuous fluorometric assays with Substrate II provide real-time and measurements. Discrepancies in reported values (e.g., due to buffer pH or temperature variations) are resolved by standardizing assay conditions per guidelines from the Beilstein Journal of Organic Chemistry, including explicit documentation of buffer composition and enzyme purity . Cross-validation with HPLC-based endpoint assays ensures reproducibility .
Advanced Research Questions
Q. How can researchers design experiments to resolve contradictions in HIV protease substrate specificity studies involving Substrate II?
- Methodological Answer : Contradictions often arise from non-standardized cleavage conditions or enzyme isoforms. A systematic approach includes:
Controlled Replicates : Use recombinant HIV protease from a single source (e.g., expressed in E. coli with uniform post-translational modifications).
Substrate Variants : Synthesize analogs (e.g., single-point mutations in Substrate II’s P1-P6 positions) to isolate specificity determinants.
Machine Learning Validation : Apply tools like HYPROSP II or SVM regression models to predict cleavage efficiency and compare with empirical data .
Q. What computational strategies integrate structural and kinetic data of Substrate II to predict novel protease inhibitors?
- Methodological Answer : Molecular dynamics simulations (e.g., GROMACS) model Substrate II-protease binding dynamics. Free energy perturbation (FEP) calculations quantify mutation effects on binding affinity. Coupling this with databases like SWISS-PROT ensures alignment with experimentally validated protease-substrate interactions .
Q. How do ethical and reproducibility standards apply to studies using Substrate II in HIV protease research?
- Methodological Answer : Adhere to the Beilstein Journal’s guidelines:
- Data Transparency : Publish full kinetic datasets in supplementary materials, including raw fluorescence traces and statistical error margins .
- Ethical Compliance : Document biosafety protocols for handling HIV protease (BSL-2+ containment) and obtain ethics approval for collaborations involving human-derived samples .
Q. What frameworks optimize the formulation of research questions for comparative studies on Substrate II analogs?
- Methodological Answer : Use the PICO framework:
- Population/Problem : HIV-1 protease.
- Intervention : Substrate II analogs with modified cleavage sites.
- Comparison : Wild-type Substrate II.
- Outcome : Cleavage efficiency ().
This structure ensures hypothesis-driven experimental design and facilitates systematic literature reviews .
Q. Data Presentation and Reproducibility
Q. How should researchers present conflicting data on Substrate II’s cleavage kinetics in publications?
- Methodological Answer : Follow the Beilstein Journal’s dual approach:
Main Text : Summarize key findings with emphasis on statistically significant trends.
Q. How can machine learning models trained on Substrate II data improve protease inhibitor discovery pipelines?
- Methodological Answer : Train models like Support Vector Machines (SVM) on datasets combining Substrate II cleavage rates and inhibitor IC50 values. Feature selection should include substrate sequence, protease mutation profiles, and solvent accessibility indices. Validate predictions using high-throughput screening (HTS) assays .
属性
IUPAC Name |
6-amino-2-[[2-[[2-[[2-[[2-[2-[[1-[2-[[2-[[2-[[2-[[2-[[6-amino-2-(3-carboxypropanoylamino)hexanoyl]amino]-3-carboxypropanoyl]amino]-3-hydroxypropanoyl]amino]-3-hydroxypropanoyl]amino]-4-methylpentanoyl]amino]-3-(4-hydroxyphenyl)propanoyl]pyrrolidine-2-carbonyl]amino]propanoylamino]-4-methylpentanoyl]amino]-3-hydroxybutanoyl]amino]-3-phenylpropanoyl]amino]-3-carboxypropanoyl]amino]hexanoic acid | |
---|---|---|
Source | PubChem | |
URL | https://pubchem.ncbi.nlm.nih.gov | |
Description | Data deposited in or computed by PubChem | |
InChI |
InChI=1S/C72H109N15O25/c1-37(2)29-46(79-67(106)52(35-88)85-68(107)53(36-89)84-65(104)50(34-58(97)98)80-61(100)44(17-10-12-26-73)76-55(92)24-25-56(93)94)62(101)83-51(32-42-20-22-43(91)23-21-42)71(110)87-28-14-19-54(87)69(108)75-39(5)60(99)78-47(30-38(3)4)66(105)86-59(40(6)90)70(109)82-48(31-41-15-8-7-9-16-41)63(102)81-49(33-57(95)96)64(103)77-45(72(111)112)18-11-13-27-74/h7-9,15-16,20-23,37-40,44-54,59,88-91H,10-14,17-19,24-36,73-74H2,1-6H3,(H,75,108)(H,76,92)(H,77,103)(H,78,99)(H,79,106)(H,80,100)(H,81,102)(H,82,109)(H,83,101)(H,84,104)(H,85,107)(H,86,105)(H,93,94)(H,95,96)(H,97,98)(H,111,112) | |
Source | PubChem | |
URL | https://pubchem.ncbi.nlm.nih.gov | |
Description | Data deposited in or computed by PubChem | |
InChI Key |
OVPKBGRKANDZBF-UHFFFAOYSA-N | |
Source | PubChem | |
URL | https://pubchem.ncbi.nlm.nih.gov | |
Description | Data deposited in or computed by PubChem | |
Canonical SMILES |
CC(C)CC(C(=O)NC(C(C)O)C(=O)NC(CC1=CC=CC=C1)C(=O)NC(CC(=O)O)C(=O)NC(CCCCN)C(=O)O)NC(=O)C(C)NC(=O)C2CCCN2C(=O)C(CC3=CC=C(C=C3)O)NC(=O)C(CC(C)C)NC(=O)C(CO)NC(=O)C(CO)NC(=O)C(CC(=O)O)NC(=O)C(CCCCN)NC(=O)CCC(=O)O | |
Source | PubChem | |
URL | https://pubchem.ncbi.nlm.nih.gov | |
Description | Data deposited in or computed by PubChem | |
Molecular Formula |
C72H109N15O25 | |
Source | PubChem | |
URL | https://pubchem.ncbi.nlm.nih.gov | |
Description | Data deposited in or computed by PubChem | |
DSSTOX Substance ID |
DTXSID40583180 | |
Record name | N~2~-(3-Carboxypropanoyl)lysyl-alpha-aspartylserylserylleucyltyrosylprolylalanylleucylthreonylphenylalanyl-alpha-aspartyllysine | |
Source | EPA DSSTox | |
URL | https://comptox.epa.gov/dashboard/DTXSID40583180 | |
Description | DSSTox provides a high quality public chemistry resource for supporting improved predictive toxicology. | |
Molecular Weight |
1584.7 g/mol | |
Source | PubChem | |
URL | https://pubchem.ncbi.nlm.nih.gov | |
Description | Data deposited in or computed by PubChem | |
CAS No. |
120944-74-3 | |
Record name | N~2~-(3-Carboxypropanoyl)lysyl-alpha-aspartylserylserylleucyltyrosylprolylalanylleucylthreonylphenylalanyl-alpha-aspartyllysine | |
Source | EPA DSSTox | |
URL | https://comptox.epa.gov/dashboard/DTXSID40583180 | |
Description | DSSTox provides a high quality public chemistry resource for supporting improved predictive toxicology. | |
体外研究产品的免责声明和信息
请注意,BenchChem 上展示的所有文章和产品信息仅供信息参考。 BenchChem 上可购买的产品专为体外研究设计,这些研究在生物体外进行。体外研究,源自拉丁语 "in glass",涉及在受控实验室环境中使用细胞或组织进行的实验。重要的是要注意,这些产品没有被归类为药物或药品,他们没有得到 FDA 的批准,用于预防、治疗或治愈任何医疗状况、疾病或疾病。我们必须强调,将这些产品以任何形式引入人类或动物的身体都是法律严格禁止的。遵守这些指南对确保研究和实验的法律和道德标准的符合性至关重要。