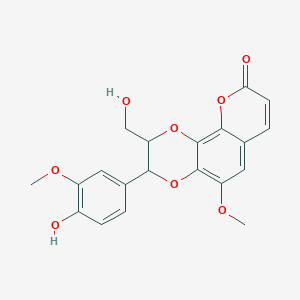
克莱米素A
描述
Cleomiscosin A is a naturally occurring coumarinolignan, primarily found in the plant Cleome viscosa L. It is a yellow crystalline compound with a bitter taste and notable medicinal properties. The chemical structure of Cleomiscosin A is characterized by a coumarin moiety linked to a lignan structure through a dioxane bridge, making it a unique member of the coumarinolignan family .
科学研究应用
Cleomiscosin A has a wide range of scientific research applications, including:
Chemistry: Used as a model compound for studying coumarinolignan synthesis and reactivity.
Biology: Investigated for its role in plant defense mechanisms and secondary metabolism.
Medicine: Exhibits anti-inflammatory, hepatoprotective, and anticancer properties.
Industry: Utilized in the development of natural product-based pharmaceuticals and nutraceuticals.
作用机制
Target of Action
Cleomiscosin A, a natural coumarinolignoid, has been found to primarily target TNF-alpha secretion in mouse peritoneal macrophages . TNF-alpha is a cell signaling protein (cytokine) involved in systemic inflammation and is one of the cytokines that make up the acute phase reaction. It is produced chiefly by activated macrophages, although it can be produced by many other cell types such as CD4+ lymphocytes, NK cells, neutrophils, mast cells, eosinophils, and neurons.
In addition, Cleomiscosin A has also been reported to inhibit the main protease of SARS-CoV-2 , and it has shown potential anti-inflammatory activities, especially against pro-inflammatory cytokines .
Mode of Action
Cleomiscosin A interacts with its targets by binding to specific residues on the active site of the targeted protein, leading to specific inhibition . For example, in the case of SARS-CoV-2, Cleomiscosin A could bind to Gln189, Glu166, Cys145, His41, and Met165 residues on the active site of the targeted protein .
Biochemical Pathways
It is known that cleomiscosin a has an inhibitory effect on the secretion of tnf-alpha , a key regulator of inflammatory responses. Therefore, it can be inferred that Cleomiscosin A may affect the pathways related to inflammation and immune response.
Pharmacokinetics
Cleomiscosin A is reported to have good pharmacokinetic properties . It is soluble, non-carcinogenic, and passes Lipinski’s rule of five, which predicts good absorption or permeation . .
Result of Action
The primary result of Cleomiscosin A’s action is the inhibition of TNF-alpha secretion in mouse peritoneal macrophages . This leads to a reduction in inflammation, given the role of TNF-alpha as a pro-inflammatory cytokine. Furthermore, Cleomiscosin A has been found to dose-dependently inhibit LDL oxidation, which suggests that it could be beneficial in preventing LDL oxidation in atherosclerotic lesions .
Action Environment
The action, efficacy, and stability of Cleomiscosin A can be influenced by various environmental factors. For instance, the compound’s solubility can be affected by the solvent used, and its stability can be influenced by storage conditions . .
生化分析
Biochemical Properties
Cleomiscosin A has been reported to exhibit activity against the secretion of TNF-alpha in mouse peritoneal macrophages . This suggests that it interacts with the biochemical pathways involving this cytokine.
Cellular Effects
Cleomiscosin A has been found to inhibit LDL oxidation in a dose-dependent manner . This suggests that it can influence cellular processes related to lipid metabolism and oxidative stress. It also exhibits inhibitory effects on pro-inflammatory cytokines in lipopolysaccharide-induced mouse endotoxaemia and carrageenan-induced mouse paw oedema models .
Molecular Mechanism
The molecular mechanism of Cleomiscosin A involves its binding interactions with biomolecules and its effects on gene expression. It has shown good binding affinity and interactions with the important triplet of arginine residues of the Thymic Stromal Lymphopoietin (TSLP), a master switch for most prevalent inflammatory allergic disorders .
Temporal Effects in Laboratory Settings
Cleomiscosin A has been found to exhibit stable effects over time in laboratory settings. For instance, in molecular dynamics simulations, the complex of Cleomiscosin A with TSLP attained stability over time with lower fluctuation of residues .
Dosage Effects in Animal Models
In animal models, the effects of Cleomiscosin A vary with dosage. For instance, it has been found to exhibit significant inhibition of pro-inflammatory cytokines at a dose of 50 mg/kg body weight in lipopolysaccharide-induced mouse endotoxaemia .
Metabolic Pathways
As a metabolite, it is likely involved in the metabolism of the plant species in which it is found .
Transport and Distribution
Given its solubility and pharmacokinetic properties , it is likely that it can be transported and distributed within cells and tissues.
准备方法
Synthetic Routes and Reaction Conditions
The total synthesis of Cleomiscosin A involves several steps, starting from readily available starting materials. One common synthetic route includes the preparation of a keto-ester intermediate, followed by reduction and subsequent hydrolysis to yield Cleomiscosin A . The key steps in the synthesis are:
- Preparation of the keto-ester from starting materials.
- Reduction of the keto-ester using lithium borohydride to form diols.
- Treatment of the diols with sulfuric acid in acetic acid to obtain Cleomiscosin A monoacetate.
- Hydrolysis of the monoacetate with sodium hydroxide to produce Cleomiscosin A .
Industrial Production Methods
Industrial production of Cleomiscosin A typically involves extraction from plant sources, particularly Cleome viscosa L. The process includes:
- Drying and pulverizing the plant material.
- Extracting the compound using solvents such as methanol or ethanol.
- Concentrating the extract and purifying Cleomiscosin A through crystallization .
化学反应分析
Types of Reactions
Cleomiscosin A undergoes various chemical reactions, including:
Oxidation: Cleomiscosin A can be oxidized to form quinone derivatives.
Reduction: Reduction reactions can convert Cleomiscosin A into dihydro derivatives.
Substitution: Cleomiscosin A can undergo substitution reactions, particularly at the hydroxyl and methoxy groups.
Common Reagents and Conditions
Oxidation: Common oxidizing agents include potassium permanganate and hydrogen peroxide.
Reduction: Lithium borohydride and sodium borohydride are commonly used reducing agents.
Substitution: Reagents such as alkyl halides and acyl chlorides are used for substitution reactions.
Major Products
The major products formed from these reactions include various derivatives of Cleomiscosin A, such as quinones, dihydro derivatives, and substituted coumarinolignans .
相似化合物的比较
Cleomiscosin A is compared with other coumarinolignans such as Cleomiscosin B and Cleomiscosin C. These compounds share similar structural features but differ in their substitution patterns and biological activities . For example:
Cleomiscosin B: Similar structure but with different hydroxyl and methoxy group positions, leading to variations in antioxidant activity.
Cleomiscosin C: Contains additional methoxy groups, resulting in enhanced antioxidant properties compared to Cleomiscosin A.
List of Similar Compounds
- Cleomiscosin B
- Cleomiscosin C
- Cosmosiine
- Pelargonidin-3-O-glucoside
Cleomiscosin A stands out due to its unique combination of coumarin and lignan structures, contributing to its diverse biological activities and potential therapeutic applications.
属性
IUPAC Name |
3-(4-hydroxy-3-methoxyphenyl)-2-(hydroxymethyl)-5-methoxy-2,3-dihydropyrano[3,2-h][1,4]benzodioxin-9-one | |
---|---|---|
Details | Computed by LexiChem 2.6.6 (PubChem release 2019.06.18) | |
Source | PubChem | |
URL | https://pubchem.ncbi.nlm.nih.gov | |
Description | Data deposited in or computed by PubChem | |
InChI |
InChI=1S/C20H18O8/c1-24-13-7-10(3-5-12(13)22)17-15(9-21)26-20-18-11(4-6-16(23)27-18)8-14(25-2)19(20)28-17/h3-8,15,17,21-22H,9H2,1-2H3 | |
Details | Computed by InChI 1.0.5 (PubChem release 2019.06.18) | |
Source | PubChem | |
URL | https://pubchem.ncbi.nlm.nih.gov | |
Description | Data deposited in or computed by PubChem | |
InChI Key |
OCBGWPJNUZMLCA-UHFFFAOYSA-N | |
Details | Computed by InChI 1.0.5 (PubChem release 2019.06.18) | |
Source | PubChem | |
URL | https://pubchem.ncbi.nlm.nih.gov | |
Description | Data deposited in or computed by PubChem | |
Canonical SMILES |
COC1=C(C=CC(=C1)C2C(OC3=C4C(=CC(=C3O2)OC)C=CC(=O)O4)CO)O | |
Details | Computed by OEChem 2.1.5 (PubChem release 2019.06.18) | |
Source | PubChem | |
URL | https://pubchem.ncbi.nlm.nih.gov | |
Description | Data deposited in or computed by PubChem | |
Molecular Formula |
C20H18O8 | |
Details | Computed by PubChem 2.1 (PubChem release 2019.06.18) | |
Source | PubChem | |
URL | https://pubchem.ncbi.nlm.nih.gov | |
Description | Data deposited in or computed by PubChem | |
Molecular Weight |
386.4 g/mol | |
Details | Computed by PubChem 2.1 (PubChem release 2021.05.07) | |
Source | PubChem | |
URL | https://pubchem.ncbi.nlm.nih.gov | |
Description | Data deposited in or computed by PubChem | |
CAS No. |
76948-72-6 | |
Record name | Cleomiscosin A | |
Source | ChemIDplus | |
URL | https://pubchem.ncbi.nlm.nih.gov/substance/?source=chemidplus&sourceid=0076948726 | |
Description | ChemIDplus is a free, web search system that provides access to the structure and nomenclature authority files used for the identification of chemical substances cited in National Library of Medicine (NLM) databases, including the TOXNET system. | |
Retrosynthesis Analysis
AI-Powered Synthesis Planning: Our tool employs the Template_relevance Pistachio, Template_relevance Bkms_metabolic, Template_relevance Pistachio_ringbreaker, Template_relevance Reaxys, Template_relevance Reaxys_biocatalysis model, leveraging a vast database of chemical reactions to predict feasible synthetic routes.
One-Step Synthesis Focus: Specifically designed for one-step synthesis, it provides concise and direct routes for your target compounds, streamlining the synthesis process.
Accurate Predictions: Utilizing the extensive PISTACHIO, BKMS_METABOLIC, PISTACHIO_RINGBREAKER, REAXYS, REAXYS_BIOCATALYSIS database, our tool offers high-accuracy predictions, reflecting the latest in chemical research and data.
Strategy Settings
Precursor scoring | Relevance Heuristic |
---|---|
Min. plausibility | 0.01 |
Model | Template_relevance |
Template Set | Pistachio/Bkms_metabolic/Pistachio_ringbreaker/Reaxys/Reaxys_biocatalysis |
Top-N result to add to graph | 6 |
Feasible Synthetic Routes
A: Cleomiscosin A has been shown to inhibit nitric oxide (NO) production in lipopolysaccharide (LPS)-activated RAW264.7 macrophages. [] This suggests that Cleomiscosin A may exert its anti-inflammatory effects by suppressing the production of pro-inflammatory mediators like NO.
A: Studies have demonstrated that Cleomiscosin A can inhibit TNF-α secretion from mouse peritoneal macrophages. [] This suggests a potential role for Cleomiscosin A in modulating inflammatory responses by targeting TNF-α.
A: Cleomiscosin A, in combination with Cleomiscosin B and C, has demonstrated significant hepatoprotective effects against carbon tetrachloride (CCl4)-induced hepatotoxicity in rat models. [] These effects are attributed to the potential of these coumarinolignoids to protect liver cells from damage.
ANone: The molecular formula of Cleomiscosin A is C20H18O7, and its molecular weight is 370.36 g/mol.
A: The structure of Cleomiscosin A has been elucidated using various spectroscopic techniques, including UV, IR, 1D and 2D NMR, and mass spectrometry (MS), including EI-MS and HR-EI-MS. [, , , , , , ]
ANone: The provided research papers primarily focus on the isolation, structural characterization, and biological activity of Cleomiscosin A. Detailed studies on its material compatibility and stability under various conditions are not available in these papers.
ANone: The provided research papers do not indicate any catalytic properties associated with Cleomiscosin A. The focus is primarily on its isolation, identification, and biological activities.
A: Yes, Cleomiscosin A has been investigated using molecular docking studies to assess its potential as an inhibitor of SARS-CoV-2 main protease (Mpro). [, , ] These studies provide insights into the binding affinity and potential interactions of Cleomiscosin A with the target protein.
A: Research suggests that the presence of a specific structural feature in Cleomiscosin A contributes to its potent tyrosinase inhibition. [] This finding highlights the importance of structural elements in determining the biological activity of Cleomiscosin A and its potential analogs.
A: While specific SAR studies for all activities of Cleomiscosin A are limited in the provided research, the isolation of regioisomers like Cleomiscosin B and malloapelins A-C suggests that structural variations can influence biological activity. [, ] Further research focused on SAR would provide a more comprehensive understanding of how structural modifications impact Cleomiscosin A's properties.
ANone: The provided research papers primarily focus on the isolation, characterization, and biological activity of Cleomiscosin A. There is limited information available regarding specific formulation strategies to improve its stability or bioavailability. Further research is needed to explore potential formulation approaches.
ANone: The provided research papers primarily focus on the isolation, characterization, and biological activities of Cleomiscosin A. Detailed studies regarding its environmental impact, degradation, and potential ecotoxicological effects are not available in these papers.
ANone: The research papers primarily focus on in vitro studies and do not provide comprehensive data on the ADME of Cleomiscosin A. Further investigations are required to understand its pharmacokinetic profile.
A: Researchers have employed LPS-stimulated RAW264.7 macrophages to investigate the anti-inflammatory properties of Cleomiscosin A in vitro. [, ] This cell line serves as a valuable model for studying inflammatory responses and the effects of potential anti-inflammatory agents.
A: Cleomiscosin A, along with Cleomiscosin B and C, has been studied in a CCl4-induced hepatotoxicity rat model to evaluate its hepatoprotective potential. [] This model is widely used to assess the ability of compounds to protect the liver from damage.
体外研究产品的免责声明和信息
请注意,BenchChem 上展示的所有文章和产品信息仅供信息参考。 BenchChem 上可购买的产品专为体外研究设计,这些研究在生物体外进行。体外研究,源自拉丁语 "in glass",涉及在受控实验室环境中使用细胞或组织进行的实验。重要的是要注意,这些产品没有被归类为药物或药品,他们没有得到 FDA 的批准,用于预防、治疗或治愈任何医疗状况、疾病或疾病。我们必须强调,将这些产品以任何形式引入人类或动物的身体都是法律严格禁止的。遵守这些指南对确保研究和实验的法律和道德标准的符合性至关重要。