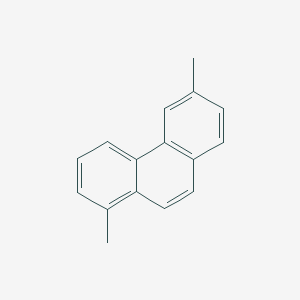
1,6-Dimethylphenanthrene
描述
1,6-Dimethylphenanthrene (C₁₆H₁₄; molecular weight: 206.30 g/mol; CAS: 20291-74-1) is a polycyclic aromatic hydrocarbon (PAH) featuring a phenanthrene backbone substituted with methyl groups at the 1- and 6-positions (Figure 1). The International Agency for Research on Cancer (IARC) has evaluated structurally related PAHs, underscoring the importance of methyl group positioning in determining biological activity and environmental impact .
准备方法
Synthetic Routes and Reaction Conditions
1,6-Dimethylphenanthrene can be synthesized through several methods. One common approach involves the cyclization of appropriate precursors under specific conditions. For example, the cyclization of 1,6-dimethyl-1,2-dihydroxybenzene with a suitable dehydrating agent can yield this compound. Another method involves the Friedel-Crafts alkylation of phenanthrene with methylating agents in the presence of a Lewis acid catalyst .
Industrial Production Methods
Industrial production of this compound typically involves the catalytic reforming of petroleum fractions. This process includes the dehydrogenation and cyclization of hydrocarbons to form aromatic compounds, including this compound. The specific conditions and catalysts used in this process can vary depending on the desired yield and purity of the final product .
化学反应分析
Types of Reactions
1,6-Dimethylphenanthrene undergoes various chemical reactions, including:
Oxidation: This compound can be oxidized to form quinones and other oxygenated derivatives.
Reduction: Reduction reactions can convert this compound to its corresponding dihydro derivatives.
Substitution: Electrophilic substitution reactions, such as nitration and sulfonation, can introduce functional groups onto the aromatic ring.
Common Reagents and Conditions
Oxidation: Common oxidizing agents include potassium permanganate and chromium trioxide.
Reduction: Reducing agents such as lithium aluminum hydride and hydrogen gas in the presence of a catalyst are often used.
Substitution: Reagents like nitric acid for nitration and sulfuric acid for sulfonation are commonly employed.
Major Products Formed
Oxidation: Quinones and other oxygenated derivatives.
Reduction: Dihydro derivatives.
Substitution: Nitro and sulfonic acid derivatives.
科学研究应用
Chemical Research Applications
1,6-DMP serves as a model compound in the study of PAH behavior due to its unique structural properties. Researchers utilize it to investigate:
- Chemical Reactivity : 1,6-DMP undergoes various reactions such as oxidation, reduction, and electrophilic substitution. For example, it can be oxidized to form quinones and other derivatives, which are crucial in understanding PAH transformations in environmental contexts.
- Synthesis of Derivatives : The compound is synthesized through methods like Friedel-Crafts alkylation and cyclization of precursors. These synthetic routes are essential for producing derivatives that may exhibit enhanced biological activity or different chemical properties.
Biological Research Applications
In biological studies, 1,6-DMP is significant for understanding the toxicity and ecological impacts of PAHs:
- Toxicity Studies : Research indicates that 1,6-DMP can activate the aryl hydrocarbon receptor (AhR), a key factor in mediating the toxic effects of PAHs. Studies have shown that methylated phenanthrenes are more potent than their unmethylated counterparts in activating AhR pathways .
- Bioaccumulation Studies : Investigations into how marine organisms like Mactra veneriformis accumulate 1,6-DMP reveal insights into ecological risks associated with oil spills. The compound's bioaccumulation potential underscores the need for monitoring PAH levels in marine environments .
Medical Research Applications
1,6-DMP is explored for its potential as a precursor in synthesizing biologically active compounds:
- Drug Development : Its structure allows for modifications that could lead to new drugs targeting specific biological pathways. The study of its derivatives provides insights into their pharmacological profiles and potential therapeutic uses .
Industrial Applications
In industrial contexts, 1,6-DMP is utilized in the production of various materials:
- Dyes and Pigments : The compound's reactivity makes it suitable for synthesizing dyes and pigments used in textiles and coatings. Its unique properties can enhance color stability and intensity.
- Polymer Manufacturing : As a component in petrochemical processes, 1,6-DMP contributes to the production of plastics and other synthetic materials. Understanding its behavior during polymerization helps optimize manufacturing processes .
Case Study 1: Toxicity Assessment
A study evaluated the toxicity of various methylated phenanthrenes using yeast bioassays. It was found that 1,6-DMP exhibited significant activation of AhR compared to other methylated derivatives. This research emphasizes the importance of structural positioning in determining toxicological profiles .
Case Study 2: Environmental Impact
Research on the bioaccumulation of 1,6-DMP in marine organisms demonstrated a consistent accumulation rate over time when exposed to contaminated environments. This study highlights potential long-term ecological risks associated with PAH contamination from oil spills .
作用机制
The mechanism of action of 1,6-dimethylphenanthrene involves its interaction with various molecular targets and pathways. In biological systems, it can undergo metabolic activation to form reactive intermediates that can bind to DNA and proteins, leading to potential toxic effects. The specific pathways involved in its metabolism include cytochrome P450 enzymes, which catalyze the oxidation of this compound to form reactive metabolites .
相似化合物的比较
Comparative Analysis with Structural Analogues
Positional Isomers of Dimethylphenanthrene
1,4-Dimethylphenanthrene (CAS: Not explicitly provided; referenced in IARC evaluations) and 4,5-Dimethylphenanthrene (CAS: Not explicitly provided) are key positional isomers. Differences in methyl group placement significantly influence physical and chemical properties:
- Crystal Packing and Density: 4,5-Dimethylphenanthrene: Exhibits a low density (1.25 g/cm³) due to inefficient molecular packing caused by steric hindrance from methyl groups. Weak van der Waals interactions (methyl-methyl) dominate its crystal structure . 1,6-Dimethylphenanthrene: No direct density data is available, but its linear substitution pattern may allow tighter packing compared to 4,5-isomers.
- Environmental Degradation: this compound serves as a parent compound in sediment samples, with predicted degradation products (e.g., hydroxylated derivatives) identified via collision cross-section (CCS) calculations . 1,4-Dimethylphenanthrene is listed in IARC reports as a compound of interest for carcinogenic risk, though specific degradation pathways are less documented .
Table 1. Comparison of Dimethylphenanthrene Isomers
Comparison with Other Methylated PAHs
Methylchrysenes (e.g., 1-Methylchrysene, CAS: 3351-22-8) and Benzo[j]fluoranthene (CAS: 205-82-3) share similarities with this compound in terms of AhR activation . However, their extended aromatic systems (four rings vs.
Table 2. Comparison with Extended PAHs
Compound | Ring System | AhR Activity | Key Application/Impact |
---|---|---|---|
This compound | 3 rings | Significant | Environmental toxicology |
Benzo[j]fluoranthene | 4 rings | Significant | Carcinogen, industrial byproduct |
1-Methylchrysene | 4 rings | Moderate | Biomarker for PAH exposure |
Functional Analogues in Pharmacology and Materials Science
- 8,8-Dimethylnaphthyridines : These anticonvulsant agents demonstrate how methyl groups enhance pharmacokinetic profiles (e.g., compound 12 in SAR studies) .
- Dihydrophenanthrenes: Derivatives like 3-amino-1-substituted-9,10-dihydrophenanthrene-2,4-dicarbonitriles exhibit antimicrobial activity, though methylation patterns differ from this compound .
Toxicological and Environmental Considerations
- AhR Activation : this compound’s AhR activity is comparable to benzo[j]fluoranthene, implicating it in dioxin-like toxicity .
- Degradation Pathways : Predicted metabolites include hydroxylated and dihydrodiol derivatives, which may exhibit higher solubility and bioavailability than the parent compound .
生物活性
1,6-Dimethylphenanthrene is a polycyclic aromatic hydrocarbon (PAH) with significant biological activity, particularly in terms of its potential mutagenic and carcinogenic properties. This article synthesizes findings from various studies to provide a comprehensive overview of its biological effects, mechanisms of action, and implications for health and environmental safety.
Chemical Structure and Properties
This compound is characterized by two methyl groups attached to the phenanthrene backbone at the 1 and 6 positions. Its chemical structure influences its solubility, reactivity, and interaction with biological systems. The compound exhibits low water solubility but high lipid solubility, affecting its bioavailability in biological systems.
Aryl Hydrocarbon Receptor (AhR) Activation
Research indicates that methylated phenanthrenes, including this compound, are more potent than unsubstituted phenanthrene in activating the AhR, a key regulator of xenobiotic metabolism. The activation of AhR leads to the induction of cytochrome P450 enzymes, which are involved in the metabolic activation of PAHs to reactive intermediates that can form DNA adducts, potentially leading to mutagenesis and carcinogenesis .
Cellular Effects and Toxicity
Studies have shown that this compound can inhibit cell growth in yeast bioassays, suggesting cytotoxic effects at certain concentrations. The dose-response relationship indicates that the position of methyl groups significantly affects the compound's potency and toxicity. For instance, while some derivatives exhibit enhanced AhR activation capabilities, others may show reduced solubility and bioactivity .
Toxicological Studies
A study assessing the developmental toxicity of alkylated PAHs found that compounds like this compound could dominate PAH contamination in various environments. These findings underscore the importance of understanding the toxicological profiles of such compounds in contaminated sites .
Biodegradation Research
Research involving microbial degradation has demonstrated that certain bacteria can metabolize this compound as a carbon source. This biodegradation pathway suggests potential environmental remediation applications but also highlights the compound's persistence in ecosystems .
Comparative Analysis of Methylated Phenanthrenes
The following table summarizes key findings related to the biological activity of various methylated phenanthrenes:
Compound | AhR Activation Potency | Cytotoxicity | Solubility | Metabolic Pathway |
---|---|---|---|---|
1-Methylphenanthrene | Moderate | Low | High | Limited microbial metabolism |
3-Methylphenanthrene | High | Moderate | Moderate | Some microbial metabolism |
4-Methylphenanthrene | Low | Low | Moderate | Limited microbial metabolism |
This compound | High | High | Low | Significant microbial metabolism |
Implications for Health and Environment
The biological activity of this compound raises concerns regarding its potential health impacts as a mutagen and carcinogen. Its ability to activate AhR pathways suggests that exposure could lead to increased risks for cancer development through mechanisms involving DNA damage and disruption of normal cellular functions. Moreover, its persistence in the environment necessitates further investigation into its ecological effects and degradation pathways.
常见问题
Basic Research Questions
Q. What are the established methods for synthesizing 1,6-dimethylphenanthrene, and how do reaction conditions influence isomer purity?
- Methodological Answer : Synthesis often involves Friedel-Crafts alkylation of phenanthrene using methyl halides. Critical parameters include temperature control (120–150°C) and catalyst selection (e.g., AlCl₃). Isomer purity is influenced by steric hindrance at substitution sites; for example, methyl groups at positions 1 and 6 reduce competing side reactions compared to ortho-substituted isomers. Post-synthesis purification via column chromatography (silica gel, hexane/ethyl acetate) is essential to isolate this compound .
Q. How does the steric hindrance of methyl groups in this compound affect its crystal packing efficiency?
- Methodological Answer : The twisted backbone and methyl groups at positions 1 and 6 create steric hindrance, leading to inefficient molecular packing. This results in low-density crystal structures (~1.20–1.25 g cm⁻³). Computational studies using density functional theory (DFT) with many-body dispersion (MBD) corrections show that weak van der Waals interactions dominate packing motifs, with methyl-methyl interactions contributing to sparse energy landscapes .
Q. What analytical techniques are recommended for quantifying this compound in environmental matrices?
- Methodological Answer : Gas chromatography-mass spectrometry (GC-MS) with selective ion monitoring (SIM) is standard. For complex matrices (e.g., sediments), use isotope dilution (e.g., phenanthrene-d10 as an internal standard). Collision cross-section (CCS) measurements via ion mobility-mass spectrometry (IM-MS), validated against in silico predictions (e.g., ISiCLE pipeline), enhance identification accuracy .
Advanced Research Questions
Q. How can evolutionary algorithms (e.g., GAtor) improve crystal structure prediction for sterically hindered compounds like this compound?
- Methodological Answer : GAtor’s niching fitness function outperforms traditional energy-based methods by penalizing oversampled regions and exploring narrow energy funnels. For this compound, evolutionary niching mitigates biases from its chiral backbone and steric hindrance, enabling discovery of the experimental structure within 10 kJ mol⁻¹ of the global minimum. Hierarchical reranking with DFT-MBD further validates stability .
Q. How should researchers address contradictions in dimethylphenanthrene maturity indices (DMPI) during organic geochemical studies?
- Methodological Answer : DMPI values (e.g., DMPI 1 and DMPI 2) can vary due to co-elution of isomers in GC-MS. To resolve contradictions:
- Use high-resolution GC columns (e.g., DB-5MS) for isomer separation.
- Apply principal component analysis (PCA) to factorize variance from isomer stability differences.
- Cross-validate with alternative indices (e.g., MPI, MPR) to confirm thermal maturity trends .
Q. What experimental designs are optimal for studying environmental degradation pathways of this compound?
- Methodological Answer : Simulate degradation via photolysis (UV light) or microbial action (e.g., Sphingomonas spp.). Monitor intermediates using:
- High-resolution LC-MS/MS for hydroxylated derivatives (e.g., 4,5-pyrenediol).
- Isotopic labeling (¹³C) to trace biodegradation efficiency.
- CCS matching via IM-MS to distinguish isobaric degradation products .
Q. How do computational methods reconcile discrepancies in the electronic properties of this compound?
- Methodological Answer : Clar’s rule predicts aromatic sextets in phenanthrene derivatives, but methyl substitution alters π-electron distribution. Use ab initio calculations (e.g., CASSCF) to map localized vs. delocalized electron densities. Compare with spectroscopic data (UV-Vis, fluorescence) to validate computational models .
Q. What strategies mitigate challenges in modeling intermolecular interactions for this compound in dense phases?
- Methodological Answer : Traditional force fields underestimate weak interactions. Apply DFT with MBD corrections to account for dispersion forces. Molecular dynamics (MD) simulations using optimized potentials (e.g., OPLS-AA/MBAR) improve predictions of melting points and solubility in nonpolar solvents .
属性
IUPAC Name |
1,6-dimethylphenanthrene | |
---|---|---|
Source | PubChem | |
URL | https://pubchem.ncbi.nlm.nih.gov | |
Description | Data deposited in or computed by PubChem | |
InChI |
InChI=1S/C16H14/c1-11-6-7-13-8-9-14-12(2)4-3-5-15(14)16(13)10-11/h3-10H,1-2H3 | |
Source | PubChem | |
URL | https://pubchem.ncbi.nlm.nih.gov | |
Description | Data deposited in or computed by PubChem | |
InChI Key |
YQMHHSZADKJARE-UHFFFAOYSA-N | |
Source | PubChem | |
URL | https://pubchem.ncbi.nlm.nih.gov | |
Description | Data deposited in or computed by PubChem | |
Canonical SMILES |
CC1=CC2=C(C=C1)C=CC3=C(C=CC=C32)C | |
Source | PubChem | |
URL | https://pubchem.ncbi.nlm.nih.gov | |
Description | Data deposited in or computed by PubChem | |
Molecular Formula |
C16H14 | |
Source | PubChem | |
URL | https://pubchem.ncbi.nlm.nih.gov | |
Description | Data deposited in or computed by PubChem | |
DSSTOX Substance ID |
DTXSID00942431 | |
Record name | 1,6-Dimethylphenanthrene | |
Source | EPA DSSTox | |
URL | https://comptox.epa.gov/dashboard/DTXSID00942431 | |
Description | DSSTox provides a high quality public chemistry resource for supporting improved predictive toxicology. | |
Molecular Weight |
206.28 g/mol | |
Source | PubChem | |
URL | https://pubchem.ncbi.nlm.nih.gov | |
Description | Data deposited in or computed by PubChem | |
CAS No. |
20291-74-1 | |
Record name | Phenanthrene, 1,6-dimethyl- | |
Source | ChemIDplus | |
URL | https://pubchem.ncbi.nlm.nih.gov/substance/?source=chemidplus&sourceid=0020291741 | |
Description | ChemIDplus is a free, web search system that provides access to the structure and nomenclature authority files used for the identification of chemical substances cited in National Library of Medicine (NLM) databases, including the TOXNET system. | |
Record name | 1,6-Dimethylphenanthrene | |
Source | EPA DSSTox | |
URL | https://comptox.epa.gov/dashboard/DTXSID00942431 | |
Description | DSSTox provides a high quality public chemistry resource for supporting improved predictive toxicology. | |
Retrosynthesis Analysis
AI-Powered Synthesis Planning: Our tool employs the Template_relevance Pistachio, Template_relevance Bkms_metabolic, Template_relevance Pistachio_ringbreaker, Template_relevance Reaxys, Template_relevance Reaxys_biocatalysis model, leveraging a vast database of chemical reactions to predict feasible synthetic routes.
One-Step Synthesis Focus: Specifically designed for one-step synthesis, it provides concise and direct routes for your target compounds, streamlining the synthesis process.
Accurate Predictions: Utilizing the extensive PISTACHIO, BKMS_METABOLIC, PISTACHIO_RINGBREAKER, REAXYS, REAXYS_BIOCATALYSIS database, our tool offers high-accuracy predictions, reflecting the latest in chemical research and data.
Strategy Settings
Precursor scoring | Relevance Heuristic |
---|---|
Min. plausibility | 0.01 |
Model | Template_relevance |
Template Set | Pistachio/Bkms_metabolic/Pistachio_ringbreaker/Reaxys/Reaxys_biocatalysis |
Top-N result to add to graph | 6 |
Feasible Synthetic Routes
体外研究产品的免责声明和信息
请注意,BenchChem 上展示的所有文章和产品信息仅供信息参考。 BenchChem 上可购买的产品专为体外研究设计,这些研究在生物体外进行。体外研究,源自拉丁语 "in glass",涉及在受控实验室环境中使用细胞或组织进行的实验。重要的是要注意,这些产品没有被归类为药物或药品,他们没有得到 FDA 的批准,用于预防、治疗或治愈任何医疗状况、疾病或疾病。我们必须强调,将这些产品以任何形式引入人类或动物的身体都是法律严格禁止的。遵守这些指南对确保研究和实验的法律和道德标准的符合性至关重要。