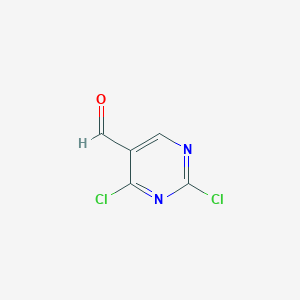
2,4-Dichloropyrimidine-5-carbaldehyde
概述
描述
2,4-Dichloropyrimidine-5-carbaldehyde is an organic compound with the molecular formula C5H2Cl2N2O. It is a white solid that is used as an intermediate in the synthesis of various chemical compounds. This compound is notable for its reactivity and versatility in organic synthesis, making it a valuable building block in the pharmaceutical and chemical industries .
准备方法
Synthetic Routes and Reaction Conditions
One common method for synthesizing 2,4-Dichloropyrimidine-5-carbaldehyde involves the reaction of 5-bromo-2,4-dichloropyrimidine with isopropyl magnesium chloride lithium chloride complex in tetrahydrofuran (THF) at -78°C . Another method includes the use of dimethylformamide (DMF) and phosphorus oxychloride (POCl3) under controlled temperature conditions .
Industrial Production Methods
Industrial production of this compound typically involves large-scale reactions using similar reagents and conditions as those used in laboratory synthesis. The process is optimized for yield and purity, often involving multiple purification steps to ensure the final product meets industrial standards .
化学反应分析
Types of Reactions
2,4-Dichloropyrimidine-5-carbaldehyde undergoes various types of chemical reactions, including:
Oxidation: The aldehyde group can be oxidized to form carboxylic acids.
Reduction: The compound can be reduced to form alcohols.
Substitution: The chlorine atoms at the 2 and 4 positions can undergo nucleophilic substitution reactions to form various derivatives.
Common Reagents and Conditions
Oxidation: Common oxidizing agents include potassium permanganate (KMnO4) and chromium trioxide (CrO3).
Reduction: Reducing agents such as sodium borohydride (NaBH4) and lithium aluminum hydride (LiAlH4) are used.
Substitution: Nucleophiles like amines and alkoxides are commonly used in substitution reactions.
Major Products
The major products formed from these reactions include carboxylic acids, alcohols, and various substituted pyrimidine derivatives .
科学研究应用
2,4-Dichloropyrimidine-5-carbaldehyde is used extensively in scientific research due to its versatility:
Chemistry: It serves as a building block for synthesizing more complex organic molecules.
Biology: It is used in the synthesis of biologically active compounds, including potential pharmaceuticals.
Medicine: The compound is a precursor in the synthesis of drugs and other therapeutic agents.
Industry: It is used in the production of agrochemicals and other industrial chemicals
作用机制
The mechanism of action of 2,4-Dichloropyrimidine-5-carbaldehyde involves its reactivity with various nucleophiles and electrophiles. The aldehyde group is highly reactive, allowing it to participate in a wide range of chemical reactions. The chlorine atoms at the 2 and 4 positions also make the compound susceptible to nucleophilic substitution, enabling the formation of diverse derivatives .
相似化合物的比较
Similar Compounds
- 4,6-Dichloropyrimidine-5-carbaldehyde
- 2,4-Dichloropyrimidine
- 2,4-Dichloro-5-methylpyrimidine
Uniqueness
2,4-Dichloropyrimidine-5-carbaldehyde is unique due to the presence of both an aldehyde group and two chlorine atoms on the pyrimidine ring. This combination of functional groups provides a high degree of reactivity and versatility, making it a valuable intermediate in organic synthesis .
属性
IUPAC Name |
2,4-dichloropyrimidine-5-carbaldehyde | |
---|---|---|
Source | PubChem | |
URL | https://pubchem.ncbi.nlm.nih.gov | |
Description | Data deposited in or computed by PubChem | |
InChI |
InChI=1S/C5H2Cl2N2O/c6-4-3(2-10)1-8-5(7)9-4/h1-2H | |
Source | PubChem | |
URL | https://pubchem.ncbi.nlm.nih.gov | |
Description | Data deposited in or computed by PubChem | |
InChI Key |
MTIXQNKVUJSOQH-UHFFFAOYSA-N | |
Source | PubChem | |
URL | https://pubchem.ncbi.nlm.nih.gov | |
Description | Data deposited in or computed by PubChem | |
Canonical SMILES |
C1=C(C(=NC(=N1)Cl)Cl)C=O | |
Source | PubChem | |
URL | https://pubchem.ncbi.nlm.nih.gov | |
Description | Data deposited in or computed by PubChem | |
Molecular Formula |
C5H2Cl2N2O | |
Source | PubChem | |
URL | https://pubchem.ncbi.nlm.nih.gov | |
Description | Data deposited in or computed by PubChem | |
DSSTOX Substance ID |
DTXSID60597108 | |
Record name | 2,4-Dichloropyrimidine-5-carbaldehyde | |
Source | EPA DSSTox | |
URL | https://comptox.epa.gov/dashboard/DTXSID60597108 | |
Description | DSSTox provides a high quality public chemistry resource for supporting improved predictive toxicology. | |
Molecular Weight |
176.99 g/mol | |
Source | PubChem | |
URL | https://pubchem.ncbi.nlm.nih.gov | |
Description | Data deposited in or computed by PubChem | |
CAS No. |
871254-61-4 | |
Record name | 2,4-Dichloropyrimidine-5-carbaldehyde | |
Source | EPA DSSTox | |
URL | https://comptox.epa.gov/dashboard/DTXSID60597108 | |
Description | DSSTox provides a high quality public chemistry resource for supporting improved predictive toxicology. | |
Synthesis routes and methods I
Procedure details
Synthesis routes and methods II
Procedure details
Retrosynthesis Analysis
AI-Powered Synthesis Planning: Our tool employs the Template_relevance Pistachio, Template_relevance Bkms_metabolic, Template_relevance Pistachio_ringbreaker, Template_relevance Reaxys, Template_relevance Reaxys_biocatalysis model, leveraging a vast database of chemical reactions to predict feasible synthetic routes.
One-Step Synthesis Focus: Specifically designed for one-step synthesis, it provides concise and direct routes for your target compounds, streamlining the synthesis process.
Accurate Predictions: Utilizing the extensive PISTACHIO, BKMS_METABOLIC, PISTACHIO_RINGBREAKER, REAXYS, REAXYS_BIOCATALYSIS database, our tool offers high-accuracy predictions, reflecting the latest in chemical research and data.
Strategy Settings
Precursor scoring | Relevance Heuristic |
---|---|
Min. plausibility | 0.01 |
Model | Template_relevance |
Template Set | Pistachio/Bkms_metabolic/Pistachio_ringbreaker/Reaxys/Reaxys_biocatalysis |
Top-N result to add to graph | 6 |
Feasible Synthetic Routes
体外研究产品的免责声明和信息
请注意,BenchChem 上展示的所有文章和产品信息仅供信息参考。 BenchChem 上可购买的产品专为体外研究设计,这些研究在生物体外进行。体外研究,源自拉丁语 "in glass",涉及在受控实验室环境中使用细胞或组织进行的实验。重要的是要注意,这些产品没有被归类为药物或药品,他们没有得到 FDA 的批准,用于预防、治疗或治愈任何医疗状况、疾病或疾病。我们必须强调,将这些产品以任何形式引入人类或动物的身体都是法律严格禁止的。遵守这些指南对确保研究和实验的法律和道德标准的符合性至关重要。