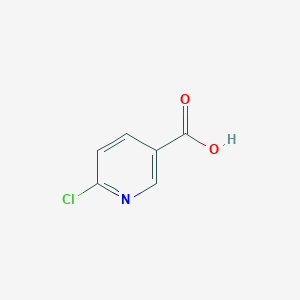
6-氯烟酸
概述
描述
6-氯烟酸是一种有机化合物,分子式为C₆H₄ClNO₂。它是烟酸的衍生物,在吡啶环的第6位上被氯原子取代。 该化合物因其在化学、生物学和工业等各个领域的应用而闻名 .
科学研究应用
作用机制
6-氯烟酸的作用机制涉及其与特定分子靶标和途径的相互作用。在新烟碱类杀虫剂的背景下,它通过干扰刺激的传递,作用于昆虫的中枢神经系统。 这是通过阻断烟碱型乙酰胆碱受体实现的,导致神经信号的破坏 .
类似化合物:
烟酸: 母体化合物,不同之处在于没有氯原子。
6-羟基烟酸: 6-氯烟酸合成的中间体。
吡虫啉: 一种具有结构相似性并靶向相同受体的烟碱类杀虫剂.
独特性: 6-氯烟酸的独特性在于氯原子的存在,它赋予了独特的化学性质和反应性。 这使其成为合成各种具有特定应用的衍生物和化合物的宝贵中间体 .
生化分析
Biochemical Properties
The exact role of 6-Chloronicotinic acid in biochemical reactions is not fully understood. It is known to interact with various enzymes, proteins, and other biomolecules. For instance, it has been reported that a bacterium, Hymenobacter latericoloratus CGMCC 16346, can degrade 64% of imidacloprid, a neonicotinoid pesticide, using maltose as a co-carbon source . The degradation process involves the transformation of imidacloprid into 6-Chloronicotinic acid .
Cellular Effects
It is known that it can cause skin irritation, serious eye irritation, and may cause respiratory irritation .
Molecular Mechanism
It is known that it can be involved in the degradation of certain pesticides, such as imidacloprid . This process involves the transformation of imidacloprid into 6-Chloronicotinic acid .
Temporal Effects in Laboratory Settings
In laboratory settings, the effects of 6-Chloronicotinic acid can change over time. For instance, it has been reported that two metabolites of imidacloprid, urea, and olefin were found to be present in the soil over a period of 60–75 days, with respective concentrations of 0.121 and 0.21 .
Dosage Effects in Animal Models
It is known that it can cause skin irritation, serious eye irritation, and may cause respiratory irritation .
Metabolic Pathways
6-Chloronicotinic acid is involved in the metabolic pathway of certain pesticides, such as imidacloprid . This process involves the transformation of imidacloprid into 6-Chloronicotinic acid .
Transport and Distribution
It is known that it can cause skin irritation, serious eye irritation, and may cause respiratory irritation .
Subcellular Localization
It is known that it can cause skin irritation, serious eye irritation, and may cause respiratory irritation .
准备方法
合成路线及反应条件: 6-氯烟酸的制备通常涉及6-羟基烟酸的氯化。该过程首先用DL-苹果酸进行环化和氨化,生成6-羟基烟酸。 然后将该中间体进行氯化反应,得到6-氯烟酸 .
工业生产方法: 在工业环境中,6-氯烟酸的合成可以通过一系列严格控制的反应来实现。例如,将6-羟基烟酸溶解在三乙胺中,然后在回流条件下加入三氯氧磷。 然后冷却反应混合物并酸化,以沉淀出所需的产物 .
化学反应分析
反应类型: 6-氯烟酸会发生各种化学反应,包括:
氧化: 它可以被氧化形成相应的羧酸。
还原: 还原反应可以将其转化为胺或其他还原形式。
常用试剂和条件:
氧化: 常用的氧化剂包括高锰酸钾和三氧化铬。
还原: 使用诸如氢化铝锂或在催化剂存在下使用氢气等还原剂。
主要生成产物:
氧化: 羧酸。
还原: 胺。
取代: 各种取代的吡啶衍生物.
相似化合物的比较
Nicotinic Acid: The parent compound, differing by the absence of the chlorine atom.
6-Hydroxynicotinic Acid: An intermediate in the synthesis of 6-chloronicotinic acid.
Imidacloprid: A neonicotinoid insecticide that shares structural similarities and targets the same receptors.
Uniqueness: 6-Chloronicotinic acid is unique due to the presence of the chlorine atom, which imparts distinct chemical properties and reactivity. This makes it a valuable intermediate in the synthesis of various derivatives and compounds with specific applications .
属性
IUPAC Name |
6-chloropyridine-3-carboxylic acid | |
---|---|---|
Source | PubChem | |
URL | https://pubchem.ncbi.nlm.nih.gov | |
Description | Data deposited in or computed by PubChem | |
InChI |
InChI=1S/C6H4ClNO2/c7-5-2-1-4(3-8-5)6(9)10/h1-3H,(H,9,10) | |
Source | PubChem | |
URL | https://pubchem.ncbi.nlm.nih.gov | |
Description | Data deposited in or computed by PubChem | |
InChI Key |
UAWMVMPAYRWUFX-UHFFFAOYSA-N | |
Source | PubChem | |
URL | https://pubchem.ncbi.nlm.nih.gov | |
Description | Data deposited in or computed by PubChem | |
Canonical SMILES |
C1=CC(=NC=C1C(=O)O)Cl | |
Source | PubChem | |
URL | https://pubchem.ncbi.nlm.nih.gov | |
Description | Data deposited in or computed by PubChem | |
Molecular Formula |
C6H4ClNO2 | |
Source | PubChem | |
URL | https://pubchem.ncbi.nlm.nih.gov | |
Description | Data deposited in or computed by PubChem | |
DSSTOX Substance ID |
DTXSID6063775 | |
Record name | 6-Chloronicotinic acid | |
Source | EPA DSSTox | |
URL | https://comptox.epa.gov/dashboard/DTXSID6063775 | |
Description | DSSTox provides a high quality public chemistry resource for supporting improved predictive toxicology. | |
Molecular Weight |
157.55 g/mol | |
Source | PubChem | |
URL | https://pubchem.ncbi.nlm.nih.gov | |
Description | Data deposited in or computed by PubChem | |
CAS No. |
5326-23-8 | |
Record name | 6-Chloronicotinic acid | |
Source | CAS Common Chemistry | |
URL | https://commonchemistry.cas.org/detail?cas_rn=5326-23-8 | |
Description | CAS Common Chemistry is an open community resource for accessing chemical information. Nearly 500,000 chemical substances from CAS REGISTRY cover areas of community interest, including common and frequently regulated chemicals, and those relevant to high school and undergraduate chemistry classes. This chemical information, curated by our expert scientists, is provided in alignment with our mission as a division of the American Chemical Society. | |
Explanation | The data from CAS Common Chemistry is provided under a CC-BY-NC 4.0 license, unless otherwise stated. | |
Record name | 6-Chloronicotinic acid | |
Source | ChemIDplus | |
URL | https://pubchem.ncbi.nlm.nih.gov/substance/?source=chemidplus&sourceid=0005326238 | |
Description | ChemIDplus is a free, web search system that provides access to the structure and nomenclature authority files used for the identification of chemical substances cited in National Library of Medicine (NLM) databases, including the TOXNET system. | |
Record name | 5326-23-8 | |
Source | DTP/NCI | |
URL | https://dtp.cancer.gov/dtpstandard/servlet/dwindex?searchtype=NSC&outputformat=html&searchlist=277 | |
Description | The NCI Development Therapeutics Program (DTP) provides services and resources to the academic and private-sector research communities worldwide to facilitate the discovery and development of new cancer therapeutic agents. | |
Explanation | Unless otherwise indicated, all text within NCI products is free of copyright and may be reused without our permission. Credit the National Cancer Institute as the source. | |
Record name | 3-Pyridinecarboxylic acid, 6-chloro- | |
Source | EPA Chemicals under the TSCA | |
URL | https://www.epa.gov/chemicals-under-tsca | |
Description | EPA Chemicals under the Toxic Substances Control Act (TSCA) collection contains information on chemicals and their regulations under TSCA, including non-confidential content from the TSCA Chemical Substance Inventory and Chemical Data Reporting. | |
Record name | 6-Chloronicotinic acid | |
Source | EPA DSSTox | |
URL | https://comptox.epa.gov/dashboard/DTXSID6063775 | |
Description | DSSTox provides a high quality public chemistry resource for supporting improved predictive toxicology. | |
Record name | 6-chloronicotinic acid | |
Source | European Chemicals Agency (ECHA) | |
URL | https://echa.europa.eu/substance-information/-/substanceinfo/100.023.819 | |
Description | The European Chemicals Agency (ECHA) is an agency of the European Union which is the driving force among regulatory authorities in implementing the EU's groundbreaking chemicals legislation for the benefit of human health and the environment as well as for innovation and competitiveness. | |
Explanation | Use of the information, documents and data from the ECHA website is subject to the terms and conditions of this Legal Notice, and subject to other binding limitations provided for under applicable law, the information, documents and data made available on the ECHA website may be reproduced, distributed and/or used, totally or in part, for non-commercial purposes provided that ECHA is acknowledged as the source: "Source: European Chemicals Agency, http://echa.europa.eu/". Such acknowledgement must be included in each copy of the material. ECHA permits and encourages organisations and individuals to create links to the ECHA website under the following cumulative conditions: Links can only be made to webpages that provide a link to the Legal Notice page. | |
Record name | 6-CHLORONICOTINIC ACID | |
Source | FDA Global Substance Registration System (GSRS) | |
URL | https://gsrs.ncats.nih.gov/ginas/app/beta/substances/99V0U0120A | |
Description | The FDA Global Substance Registration System (GSRS) enables the efficient and accurate exchange of information on what substances are in regulated products. Instead of relying on names, which vary across regulatory domains, countries, and regions, the GSRS knowledge base makes it possible for substances to be defined by standardized, scientific descriptions. | |
Explanation | Unless otherwise noted, the contents of the FDA website (www.fda.gov), both text and graphics, are not copyrighted. They are in the public domain and may be republished, reprinted and otherwise used freely by anyone without the need to obtain permission from FDA. Credit to the U.S. Food and Drug Administration as the source is appreciated but not required. | |
Synthesis routes and methods I
Procedure details
Synthesis routes and methods II
Procedure details
Synthesis routes and methods III
Procedure details
Synthesis routes and methods IV
Procedure details
Retrosynthesis Analysis
AI-Powered Synthesis Planning: Our tool employs the Template_relevance Pistachio, Template_relevance Bkms_metabolic, Template_relevance Pistachio_ringbreaker, Template_relevance Reaxys, Template_relevance Reaxys_biocatalysis model, leveraging a vast database of chemical reactions to predict feasible synthetic routes.
One-Step Synthesis Focus: Specifically designed for one-step synthesis, it provides concise and direct routes for your target compounds, streamlining the synthesis process.
Accurate Predictions: Utilizing the extensive PISTACHIO, BKMS_METABOLIC, PISTACHIO_RINGBREAKER, REAXYS, REAXYS_BIOCATALYSIS database, our tool offers high-accuracy predictions, reflecting the latest in chemical research and data.
Strategy Settings
Precursor scoring | Relevance Heuristic |
---|---|
Min. plausibility | 0.01 |
Model | Template_relevance |
Template Set | Pistachio/Bkms_metabolic/Pistachio_ringbreaker/Reaxys/Reaxys_biocatalysis |
Top-N result to add to graph | 6 |
Feasible Synthetic Routes
Q1: What is the primary source of 6-Chloronicotinic acid in the environment?
A1: 6-Chloronicotinic acid is a major metabolite of several widely used neonicotinoid insecticides, including imidacloprid, acetamiprid, and nitenpyram. These insecticides are used on a variety of crops and can persist in soil and water, leading to the formation and accumulation of 6-CNA. [, , , ]
Q2: Does 6-Chloronicotinic acid interact with the nicotinic acetylcholine receptor (nAChR) like its parent neonicotinoid compounds?
A2: While 6-CNA shares structural similarities with neonicotinoids, studies show it has significantly lower affinity for insect nAChRs. [, ] Experiments with honeybees demonstrated that 6-CNA did not induce inward currents in neurons expressing nAChRs even at high concentrations (3 mM). In contrast, imidacloprid and certain other metabolites exhibited potent agonistic activity on these receptors. []
Q3: Is there evidence that 6-CNA acts as a chemosensitizer in some organisms?
A3: Yes, research on amphipods (Gammarus fossarum) revealed that 6-CNA can inhibit the multixenobiotic resistance (MXR) defense system at low concentrations. This system, mediated by efflux transporters, normally protects organisms from xenobiotics. [] Inhibition of MXR by 6-CNA could potentially lead to increased cellular uptake and toxicity of other contaminants present in the environment.
Q4: What is the molecular formula and weight of 6-Chloronicotinic acid?
A4: The molecular formula of 6-Chloronicotinic acid is C6H4ClNO2, and its molecular weight is 157.55 g/mol.
Q5: Are there spectroscopic methods available for 6-Chloronicotinic acid identification and quantification?
A5: Yes, several spectroscopic methods have been employed for 6-CNA analysis, including:
- 1H NMR: This technique can monitor the photocatalytic degradation of 6-CNA, providing insights into degradation kinetics and potential intermediates. []
- FTIR: This method has been used to track the degradation of specific functional groups within 6-CNA during photocatalysis. []
Q6: What analytical techniques are commonly employed for the detection and quantification of 6-Chloronicotinic acid in environmental and biological matrices?
A6: Several analytical techniques have been developed and validated for 6-CNA determination, including:
- High-Performance Liquid Chromatography (HPLC): Often coupled with diode-array detection (DAD) or mass spectrometry (MS), HPLC is widely used for separating and quantifying 6-CNA in various matrices, including sweet cherry samples, environmental samples, and urine. [, , , , , , ]
- Gas Chromatography-Mass Spectrometry (GC-MS): This technique, often coupled with derivatization steps, offers high sensitivity and selectivity for 6-CNA analysis in complex matrices like coffee, urine, and industrial water. [, , ]
- Ion Chromatography (IC): Coupled with online photoinduced fluorescence detection, IC provides a sensitive and cost-effective approach for simultaneous determination of 6-CNA and its parent neonicotinoids in environmental samples. [, ]
- Fluorescence Polarization Immunoassay (FPIA): While less sensitive than chromatographic techniques, FPIA offers a rapid and simple method for screening 6-CNA in agricultural, environmental, and biological samples. []
Q7: How persistent is 6-Chloronicotinic acid in the environment?
A7: The persistence of 6-CNA varies depending on environmental factors such as soil type, pH, and microbial activity. While some studies indicate it can persist for extended periods, others report relatively rapid degradation. [, , ] Research is ongoing to better understand its environmental fate and potential for bioaccumulation.
Q8: Has 6-Chloronicotinic acid been detected in human samples?
A8: Yes, 6-CNA has been detected in the urine of individuals exposed to neonicotinoid insecticides. [, , ] These findings highlight the potential for human exposure to this metabolite through various pathways, including the consumption of contaminated food and beverages.
Q9: What is the known toxicity profile of 6-Chloronicotinic acid in mammals?
A9: While 6-CNA is generally considered less toxic than its parent neonicotinoids, research on its specific toxicological effects is still developing. Some studies suggest it may have neurotoxic effects, but more research is needed to fully understand its potential risks to human health. [, ]
Q10: Are there microorganisms capable of degrading 6-Chloronicotinic acid?
A10: Yes, several bacterial strains have been identified that can degrade 6-CNA. These include:* Bradyrhizobiaceae strain SG-6C: This strain hydrolytically dechlorinates 6-CNA to 6-hydroxynicotinic acid, further metabolized via the nicotinic acid pathway. []* Leifsonia sp. PC-21: This strain cometabolizes imidacloprid, with 6-CNA as an intermediate that undergoes further degradation. []* Bacillus alkalinitrilicus: This bacterium has been shown to enhance the degradation of imidacloprid in soil, with 6-CNA identified as a major metabolite. []* Bacillus aerophilus: Similar to B. alkalinitrilicus, this bacterium exhibits imidacloprid degradation capabilities, producing 6-CNA as a metabolite. []
Q11: Can photocatalysis be used to degrade 6-Chloronicotinic acid?
A11: Yes, research demonstrates that 6-CNA can be effectively degraded by photocatalysis using TiO2 as a catalyst under UV irradiation. [, , ] This method holds promise for the removal of 6-CNA from contaminated water sources.
体外研究产品的免责声明和信息
请注意,BenchChem 上展示的所有文章和产品信息仅供信息参考。 BenchChem 上可购买的产品专为体外研究设计,这些研究在生物体外进行。体外研究,源自拉丁语 "in glass",涉及在受控实验室环境中使用细胞或组织进行的实验。重要的是要注意,这些产品没有被归类为药物或药品,他们没有得到 FDA 的批准,用于预防、治疗或治愈任何医疗状况、疾病或疾病。我们必须强调,将这些产品以任何形式引入人类或动物的身体都是法律严格禁止的。遵守这些指南对确保研究和实验的法律和道德标准的符合性至关重要。