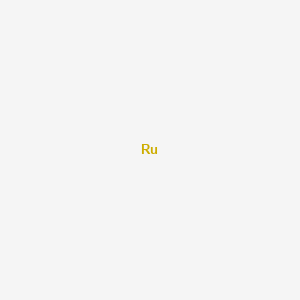
钌
概述
描述
Ruthenium is a transition metal belonging to the platinum group of the periodic table. It is represented by the symbol Ru and has an atomic number of 44. Ruthenium is a rare, silvery-white metal that is hard and brittle. It was discovered by Karl Ernst Claus in 1844 and named after Ruthenia, the Latin name for Russia . Ruthenium is primarily found in ores containing other platinum group metals and is often used as an alloying agent to improve the hardness and corrosion resistance of other metals .
科学研究应用
钌在各个领域都有广泛的科学研究应用:
化学:
生物学和医学:
工业:
作用机制
钌化合物的作用机制取决于其具体的应用:
抗癌活性:
- 钌配合物可以与 DNA 结合,干扰其复制和转录。 这导致抑制肿瘤细胞生长和增殖 .
- 一些钌化合物靶向参与癌细胞代谢的特定酶,进一步增强其抗癌作用 .
催化活性:
安全和危害
未来方向
Ruthenium-based materials for OER and HER are very promising because of their prominent catalytic activity, pH-universal application, the cheapest price among the precious metal family, and so on . The main aim here is to shed some light on the design and construction of emerging catalysts for energy storage and conversion technologies .
生化分析
Biochemical Properties
Ruthenium on carbon plays a significant role in biochemical reactions. It has been used as a catalyst in various chemical transformations, including hydrogenation . Ruthenium catalysts can selectively hydrogenate carbon-carbon triple bonds in alkynes to alkenes (partial hydrogenation) or to alkanes (complete hydrogenation) . These interactions are primarily due to the ability of Ruthenium to form complexes with various biomolecules .
Cellular Effects
Ruthenium complexes have shown remarkable antitumor activity among numerous metal compounds studied . They possess various advantages over platinum drugs, such as potent efficacy, low toxicity, less drug resistance . Ruthenium complexes act on DNA, mitochondria, and endoplasmic reticulum of cells, as well as signaling pathways that induce tumor cell apoptosis, autophagy, and inhibition of angiogenesis .
Molecular Mechanism
Ruthenium complexes exert their effects at the molecular level through various mechanisms. For instance, one study revealed that Ruthenium complex induced DNA phase separation in living cells . Another study showed that a Ruthenium complex with triazolopyrimidine derivatives demonstrated potential for use as anticancer agents by maintaining the toxic effect on MCF-7 and HeLa cells .
Temporal Effects in Laboratory Settings
In laboratory settings, the effects of Ruthenium on carbon can change over time. For instance, a study on the kinetics and photochemistry of Ruthenium bisbipyridine diacetonitrile complexes showed that upon green light irradiation, the non-toxic ligand is selectively cleaved off, thereby releasing a phototoxic Ruthenium-based photoproduct capable notably of binding to nuclear DNA and triggering DNA damage and apoptosis within 24–48 hours .
Dosage Effects in Animal Models
The effects of Ruthenium on carbon vary with different dosages in animal models. For instance, a study showed that a Ruthenium complex encapsulated with pluronic® F-127 induced oncosis in A549 cells . Another study showed that a Ruthenium complex exhibited excellent anti-cancer activity, with low toxicity, across a large panel of human pancreatic patient-derived xenografts .
Metabolic Pathways
Ruthenium on carbon is involved in various metabolic pathways. For instance, a study showed that Ruthenium complexes can trigger oxidative stress, induce mitochondrial membrane potential depolarization, and drastically reduce intracellular ATP levels .
Transport and Distribution
Ruthenium on carbon is transported and distributed within cells and tissues in various ways. For instance, a study showed that a Ruthenium complex was capable of inhibiting cellular oxidative phosphorylation (OXPHOS) function in vitro .
Subcellular Localization
The subcellular localization of Ruthenium on carbon and its effects on activity or function have been studied. For instance, a study showed that a Ruthenium complex was localized in the lysosomes of cells . Another study showed that a Ruthenium complex was capable of inducing DNA phase separation in living cells .
准备方法
合成路线和反应条件: 钌可以通过多种合成路线制备。一种常见的方法是利用流动反应器用硼氢化钠还原硝基硝酸钌。 这种方法可以合成尺寸可调的钌纳米粒子 。 另一种方法是使用纳米材料负载的钌催化剂,这些催化剂是使用载体材料、前体和钌纳米粒子制备的 .
工业生产方法: 在工业环境中,钌通常从铂矿石中提取。提取过程涉及多个步骤,包括破碎矿石、浮选和熔炼。然后用王水处理所得精矿以溶解铂族金属。 钌通过一系列化学反应(包括沉淀和溶剂萃取)从溶液中分离出来 .
化学反应分析
反应类型: 钌经历各种类型的化学反应,包括氧化、还原和取代反应。 它以其催化性能而闻名,尤其是在加氢和氢解反应中 。 钌还可以参与氧化反应,例如使用四氧化钌将醇氧化为羧酸 .
常见试剂和条件:
主要产品:
相似化合物的比较
钌与其他铂族金属(如锇、铱和铂)具有相似之处。它也有一些独特的特性使其与众不同:
类似化合物:
钌的独特性:
总之,钌是一种用途广泛且宝贵的金属,在化学、生物学、医学和工业中具有广泛的应用。它独特的特性和催化能力使其成为科学研究和工业过程的重要化合物。
属性
IUPAC Name |
ruthenium | |
---|---|---|
Source | PubChem | |
URL | https://pubchem.ncbi.nlm.nih.gov | |
Description | Data deposited in or computed by PubChem | |
InChI |
InChI=1S/Ru | |
Source | PubChem | |
URL | https://pubchem.ncbi.nlm.nih.gov | |
Description | Data deposited in or computed by PubChem | |
InChI Key |
KJTLSVCANCCWHF-UHFFFAOYSA-N | |
Source | PubChem | |
URL | https://pubchem.ncbi.nlm.nih.gov | |
Description | Data deposited in or computed by PubChem | |
Canonical SMILES |
[Ru] | |
Source | PubChem | |
URL | https://pubchem.ncbi.nlm.nih.gov | |
Description | Data deposited in or computed by PubChem | |
Molecular Formula |
Ru | |
Source | PubChem | |
URL | https://pubchem.ncbi.nlm.nih.gov | |
Description | Data deposited in or computed by PubChem | |
DSSTOX Substance ID |
DTXSID9064687 | |
Record name | Ruthenium | |
Source | EPA DSSTox | |
URL | https://comptox.epa.gov/dashboard/DTXSID9064687 | |
Description | DSSTox provides a high quality public chemistry resource for supporting improved predictive toxicology. | |
Molecular Weight |
101.1 g/mol | |
Source | PubChem | |
URL | https://pubchem.ncbi.nlm.nih.gov | |
Description | Data deposited in or computed by PubChem | |
Physical Description |
Liquid, Other Solid, Lustrous metal; [Merck Index], Solid | |
Record name | Ruthenium | |
Source | EPA Chemicals under the TSCA | |
URL | https://www.epa.gov/chemicals-under-tsca | |
Description | EPA Chemicals under the Toxic Substances Control Act (TSCA) collection contains information on chemicals and their regulations under TSCA, including non-confidential content from the TSCA Chemical Substance Inventory and Chemical Data Reporting. | |
Record name | Ruthenium | |
Source | Haz-Map, Information on Hazardous Chemicals and Occupational Diseases | |
URL | https://haz-map.com/Agents/73 | |
Description | Haz-Map® is an occupational health database designed for health and safety professionals and for consumers seeking information about the adverse effects of workplace exposures to chemical and biological agents. | |
Explanation | Copyright (c) 2022 Haz-Map(R). All rights reserved. Unless otherwise indicated, all materials from Haz-Map are copyrighted by Haz-Map(R). No part of these materials, either text or image may be used for any purpose other than for personal use. Therefore, reproduction, modification, storage in a retrieval system or retransmission, in any form or by any means, electronic, mechanical or otherwise, for reasons other than personal use, is strictly prohibited without prior written permission. | |
Record name | Ruthenium | |
Source | Human Metabolome Database (HMDB) | |
URL | http://www.hmdb.ca/metabolites/HMDB0013668 | |
Description | The Human Metabolome Database (HMDB) is a freely available electronic database containing detailed information about small molecule metabolites found in the human body. | |
Explanation | HMDB is offered to the public as a freely available resource. Use and re-distribution of the data, in whole or in part, for commercial purposes requires explicit permission of the authors and explicit acknowledgment of the source material (HMDB) and the original publication (see the HMDB citing page). We ask that users who download significant portions of the database cite the HMDB paper in any resulting publications. | |
CAS No. |
7440-18-8 | |
Record name | Ruthenium | |
Source | CAS Common Chemistry | |
URL | https://commonchemistry.cas.org/detail?cas_rn=7440-18-8 | |
Description | CAS Common Chemistry is an open community resource for accessing chemical information. Nearly 500,000 chemical substances from CAS REGISTRY cover areas of community interest, including common and frequently regulated chemicals, and those relevant to high school and undergraduate chemistry classes. This chemical information, curated by our expert scientists, is provided in alignment with our mission as a division of the American Chemical Society. | |
Explanation | The data from CAS Common Chemistry is provided under a CC-BY-NC 4.0 license, unless otherwise stated. | |
Record name | Ruthenium | |
Source | ChemIDplus | |
URL | https://pubchem.ncbi.nlm.nih.gov/substance/?source=chemidplus&sourceid=0007440188 | |
Description | ChemIDplus is a free, web search system that provides access to the structure and nomenclature authority files used for the identification of chemical substances cited in National Library of Medicine (NLM) databases, including the TOXNET system. | |
Record name | Ruthenium | |
Source | EPA Chemicals under the TSCA | |
URL | https://www.epa.gov/chemicals-under-tsca | |
Description | EPA Chemicals under the Toxic Substances Control Act (TSCA) collection contains information on chemicals and their regulations under TSCA, including non-confidential content from the TSCA Chemical Substance Inventory and Chemical Data Reporting. | |
Record name | Ruthenium | |
Source | EPA DSSTox | |
URL | https://comptox.epa.gov/dashboard/DTXSID9064687 | |
Description | DSSTox provides a high quality public chemistry resource for supporting improved predictive toxicology. | |
Record name | Ruthenium | |
Source | European Chemicals Agency (ECHA) | |
URL | https://echa.europa.eu/substance-information/-/substanceinfo/100.028.297 | |
Description | The European Chemicals Agency (ECHA) is an agency of the European Union which is the driving force among regulatory authorities in implementing the EU's groundbreaking chemicals legislation for the benefit of human health and the environment as well as for innovation and competitiveness. | |
Explanation | Use of the information, documents and data from the ECHA website is subject to the terms and conditions of this Legal Notice, and subject to other binding limitations provided for under applicable law, the information, documents and data made available on the ECHA website may be reproduced, distributed and/or used, totally or in part, for non-commercial purposes provided that ECHA is acknowledged as the source: "Source: European Chemicals Agency, http://echa.europa.eu/". Such acknowledgement must be included in each copy of the material. ECHA permits and encourages organisations and individuals to create links to the ECHA website under the following cumulative conditions: Links can only be made to webpages that provide a link to the Legal Notice page. | |
Record name | RUTHENIUM | |
Source | FDA Global Substance Registration System (GSRS) | |
URL | https://gsrs.ncats.nih.gov/ginas/app/beta/substances/7UI0TKC3U5 | |
Description | The FDA Global Substance Registration System (GSRS) enables the efficient and accurate exchange of information on what substances are in regulated products. Instead of relying on names, which vary across regulatory domains, countries, and regions, the GSRS knowledge base makes it possible for substances to be defined by standardized, scientific descriptions. | |
Explanation | Unless otherwise noted, the contents of the FDA website (www.fda.gov), both text and graphics, are not copyrighted. They are in the public domain and may be republished, reprinted and otherwise used freely by anyone without the need to obtain permission from FDA. Credit to the U.S. Food and Drug Administration as the source is appreciated but not required. | |
Record name | Ruthenium | |
Source | Human Metabolome Database (HMDB) | |
URL | http://www.hmdb.ca/metabolites/HMDB0013668 | |
Description | The Human Metabolome Database (HMDB) is a freely available electronic database containing detailed information about small molecule metabolites found in the human body. | |
Explanation | HMDB is offered to the public as a freely available resource. Use and re-distribution of the data, in whole or in part, for commercial purposes requires explicit permission of the authors and explicit acknowledgment of the source material (HMDB) and the original publication (see the HMDB citing page). We ask that users who download significant portions of the database cite the HMDB paper in any resulting publications. | |
Melting Point |
2334 °C | |
Record name | Ruthenium | |
Source | Human Metabolome Database (HMDB) | |
URL | http://www.hmdb.ca/metabolites/HMDB0013668 | |
Description | The Human Metabolome Database (HMDB) is a freely available electronic database containing detailed information about small molecule metabolites found in the human body. | |
Explanation | HMDB is offered to the public as a freely available resource. Use and re-distribution of the data, in whole or in part, for commercial purposes requires explicit permission of the authors and explicit acknowledgment of the source material (HMDB) and the original publication (see the HMDB citing page). We ask that users who download significant portions of the database cite the HMDB paper in any resulting publications. | |
Synthesis routes and methods
Procedure details
A: Ruthenium complexes, particularly those containing nonsteroidal anti-inflammatory drug (NSAID) derived ligands, have demonstrated interactions with serum proteins like transferrin and albumin []. These interactions differ from those observed with precursor complexes like [Ru2(O2CCH3)4Cl] []. Furthermore, ruthenium(III) complexes with ligands like 3-(benzothiazol-2-yliminomethyl)-naphthalen-2-ol exhibit DNA binding affinity and induce DNA cleavage []. This interaction with DNA and potential for DNA damage contributes to their cytotoxic activity against cancer cell lines like human cervical cancer and human laryngeal epithelial carcinoma cells [].
A: Characterization of ruthenium complexes often involves techniques like X-ray crystallography, infrared spectroscopy (IR), ultraviolet-visible spectroscopy (UV-Vis), electron paramagnetic resonance (EPR), and cyclic voltammetry (CV) []. For instance, ruthenium(III) complexes with bulky triazolopyrimidine ligands like 5,7-ditertbutyl-1,2,4-triazolo[1,5-a]pyrimidine (dbtp) have been structurally characterized. Examples include trans-[RuCl(3)(H(2)O)(dbtp)(2)] and mer-[RuCl(3)(dbtp)(3)]·0.815OCMe(2), which exhibit distorted octahedral geometries []. These characterization techniques provide valuable insights into the structure, bonding, and electronic properties of ruthenium complexes.
A: Ruthenium, often employed as a thin film in microelectronics, presents challenges due to its high resistivity []. To address this, researchers are exploring direct copper electroplating on ruthenium surfaces []. This approach requires careful optimization of plating conditions, including current density, bath composition, and pretreatment to control copper nucleation and achieve uniform, low-resistance copper films on ruthenium [].
A: * Fischer-Tropsch Synthesis: Ruthenium catalysts excel in the Fischer-Tropsch synthesis, converting synthesis gas (CO and H2) into hydrocarbons []. The support material significantly impacts the catalyst's performance. For example, a ruthenium catalyst on sodium Y zeolite demonstrated higher chain growth, lower methane selectivity, and a higher olefin-to-paraffin ratio compared to a conventional alumina-supported catalyst []. * Hydrogenation Reactions: Ruthenium complexes, including those with CNN- and PNN-pincer ligands, are highly effective catalysts for ester hydrogenation []. These complexes often undergo dehydroalkylation under reaction conditions, forming ruthenium(0) species with imine functionalities, which are the proposed active catalytic species []. * CO2 Hydrogenation: Ruthenium N-heterocyclic carbene (NHC) complexes are promising catalysts for CO2 hydrogenation to amides []. Researchers are developing tailored hybrid materials by anchoring these complexes onto silica surfaces, aiming to combine the advantages of homogeneous and heterogeneous catalysts [].
A: Computational techniques like Density Functional Theory (DFT) are crucial for understanding the electronic structure and properties of ruthenium complexes []. For example, DFT calculations were used to study a ruthenium(II) imine complex anchored to a silica surface []. These calculations helped interpret experimental spectroscopic data and provided insights into the electronic transitions responsible for the observed UV-Vis spectra [].
A: In ruthenium(III) dithiocarbamate complexes, the nature of the dithiocarbamate ligand significantly influences cytotoxicity []. For instance, a complex with a piperidine substituent, tris(piperidinedithiocarbamato)ruthenium(III), exhibited higher cytotoxicity compared to complexes containing phenyl rings in their ligands []. This difference highlights the importance of ligand structure in modulating the biological activity of ruthenium complexes.
ANone: While not directly addressed in the provided papers, formulation strategies such as encapsulation in nanoparticles or conjugation to biocompatible polymers are commonly investigated to improve the stability, solubility, and bioavailability of metal-based drugs, including ruthenium complexes.
A: While SHE regulations are not explicitly discussed in the provided papers, responsible research practices necessitate considering the environmental impact of ruthenium compounds. This includes understanding their potential toxicity, degradation pathways, and developing strategies for their safe handling, disposal, and recycling [, ].
A: Recycling and waste management of ruthenium, a precious metal, are crucial due to its economic value and potential environmental impact []. Various methods, including hydrometallurgical and pyrometallurgical processes, are employed to recover ruthenium from spent catalysts and other sources [].
A: The discovery of ruthenium complexes with remarkable anticancer activity, such as KP1019 and NAMI-A, marked a significant milestone [, ]. These findings sparked intense research into ruthenium-based metallodrugs as potential alternatives to platinum-based chemotherapy agents [, ].
A: Ruthenium research exemplifies the power of interdisciplinary collaboration, bringing together chemists, biologists, material scientists, and engineers []. For instance, the development of ruthenium-based anticancer drugs requires expertise in inorganic synthesis, medicinal chemistry, pharmacology, and oncology [, ].
体外研究产品的免责声明和信息
请注意,BenchChem 上展示的所有文章和产品信息仅供信息参考。 BenchChem 上可购买的产品专为体外研究设计,这些研究在生物体外进行。体外研究,源自拉丁语 "in glass",涉及在受控实验室环境中使用细胞或组织进行的实验。重要的是要注意,这些产品没有被归类为药物或药品,他们没有得到 FDA 的批准,用于预防、治疗或治愈任何医疗状况、疾病或疾病。我们必须强调,将这些产品以任何形式引入人类或动物的身体都是法律严格禁止的。遵守这些指南对确保研究和实验的法律和道德标准的符合性至关重要。