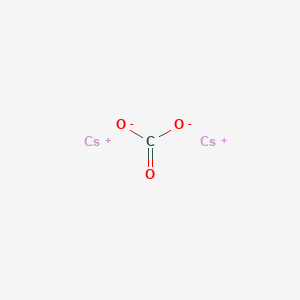
Cesium carbonate
描述
Cesium carbonate is a chemical compound with the formula Cs₂CO₃. It is a white crystalline solid that is highly soluble in polar solvents such as water, ethanol, and dimethylformamide. This compound is known for its use as a base in organic synthesis and has applications in various fields, including energy conversion .
Synthetic Routes and Reaction Conditions:
- this compound can be prepared by the thermal decomposition of cesium oxalate. When heated, cesium oxalate decomposes to form this compound and carbon monoxide:
Thermal Decomposition: Cs2C2O4→Cs2CO3+CO
Another method involves reacting cesium hydroxide with carbon dioxide:Reaction with Carbon Dioxide: 2CsOH+CO2→Cs2CO3+H2O
Industrial Production Methods:
Alkaline Process: This method involves grinding cesium ore to a fine powder, mixing it with sodium carbonate and sodium chloride, and then heating the mixture in a high-temperature furnace.
Acid Process: In this method, cesium ore is ground and mixed with water and sulfuric acid.
Types of Reactions:
N-Alkylation: this compound facilitates the N-alkylation of compounds such as sulfonamides, amines, β-lactams, indoles, and heterocyclic compounds.
Substitution: It is used in various substitution reactions, including the Suzuki coupling and Buchwald-Hartwig amination reactions.
Common Reagents and Conditions:
Reagents: Sodium tetrachloroaurate, dimethyl sulfoxide, dimethylformamide, and various organic solvents.
Conditions: Reactions typically occur at room temperature or under mild heating conditions.
Major Products:
Ketones and Aldehydes: From the oxidation of alcohols.
N-Alkylated Compounds: From the N-alkylation reactions.
科学研究应用
Cesium carbonate has a wide range of applications in scientific research:
Organic Synthesis: It is used as a mild inorganic base in various organic transformations, including C, N, and O alkylation and arylation reactions.
Energy Conversion: this compound is used in the development of energy conversion devices due to its unique properties.
Material Science: It is employed in the synthesis of phosphazene derivatives and other advanced materials.
作用机制
Cesium carbonate acts as a base in organic reactions, facilitating the deprotonation of substrates and promoting various chemical transformations. Its large cationic radius and high solubility in organic solvents enhance its reactivity and efficiency in these reactions . The compound’s ability to form stable ion pairs in dipolar aprotic solvents further contributes to its effectiveness .
相似化合物的比较
- Lithium Carbonate
- Sodium Carbonate
- Potassium Carbonate
- Rubidium Carbonate
Comparison:
- Solubility: Cesium carbonate has higher solubility in organic solvents compared to other carbonates like potassium carbonate and sodium carbonate .
- Reactivity: The large cationic radius of cesium ions makes this compound more reactive and efficient in promoting organic reactions .
- Applications: While other carbonates are also used as bases in organic synthesis, this compound’s unique properties make it particularly valuable in specific applications such as energy conversion and advanced material synthesis .
This compound stands out due to its high solubility, reactivity, and versatility in various scientific and industrial applications. Its unique properties make it a valuable compound in the field of chemistry and beyond.
属性
CAS 编号 |
534-17-8 |
---|---|
分子式 |
CH2CsO3 |
分子量 |
194.930 g/mol |
IUPAC 名称 |
dicesium;carbonate |
InChI |
InChI=1S/CH2O3.Cs/c2-1(3)4;/h(H2,2,3,4); |
InChI 键 |
UHKPOGGUWJGGID-UHFFFAOYSA-N |
SMILES |
C(=O)([O-])[O-].[Cs+].[Cs+] |
规范 SMILES |
C(=O)(O)O.[Cs] |
29703-01-3 534-17-8 |
|
物理描述 |
DryPowder, WetSolid |
Pictograms |
Corrosive; Irritant; Health Hazard |
相关CAS编号 |
29703-01-3 |
同义词 |
Carbonic Acid Dicesium Salt; Cesium Carbonate (Cs2CO3); Cesium Carbonate; Dicesium Carbonate; |
产品来源 |
United States |
Synthesis routes and methods
Procedure details
Retrosynthesis Analysis
AI-Powered Synthesis Planning: Our tool employs the Template_relevance Pistachio, Template_relevance Bkms_metabolic, Template_relevance Pistachio_ringbreaker, Template_relevance Reaxys, Template_relevance Reaxys_biocatalysis model, leveraging a vast database of chemical reactions to predict feasible synthetic routes.
One-Step Synthesis Focus: Specifically designed for one-step synthesis, it provides concise and direct routes for your target compounds, streamlining the synthesis process.
Accurate Predictions: Utilizing the extensive PISTACHIO, BKMS_METABOLIC, PISTACHIO_RINGBREAKER, REAXYS, REAXYS_BIOCATALYSIS database, our tool offers high-accuracy predictions, reflecting the latest in chemical research and data.
Strategy Settings
Precursor scoring | Relevance Heuristic |
---|---|
Min. plausibility | 0.01 |
Model | Template_relevance |
Template Set | Pistachio/Bkms_metabolic/Pistachio_ringbreaker/Reaxys/Reaxys_biocatalysis |
Top-N result to add to graph | 6 |
Feasible Synthetic Routes
体外研究产品的免责声明和信息
请注意,BenchChem 上展示的所有文章和产品信息仅供信息参考。 BenchChem 上可购买的产品专为体外研究设计,这些研究在生物体外进行。体外研究,源自拉丁语 "in glass",涉及在受控实验室环境中使用细胞或组织进行的实验。重要的是要注意,这些产品没有被归类为药物或药品,他们没有得到 FDA 的批准,用于预防、治疗或治愈任何医疗状况、疾病或疾病。我们必须强调,将这些产品以任何形式引入人类或动物的身体都是法律严格禁止的。遵守这些指南对确保研究和实验的法律和道德标准的符合性至关重要。