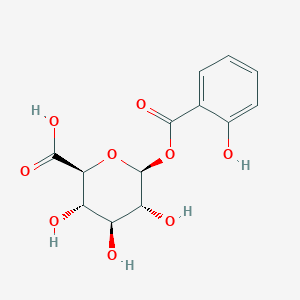
(2S,3S,4S,5R,6S)-3,4,5-三羟基-6-(2-羟基苯甲酰)氧杂环-2-羧酸
描述
(2S,3S,4S,5R,6S)-3,4,5-trihydroxy-6-(2-hydroxybenzoyl)oxyoxane-2-carboxylic acid, more commonly known as THB-6-OH, is a naturally occurring compound found in plants and fungi. It is a major component of the flavonoid family and has been studied extensively for its potential therapeutic applications. THB-6-OH has been found to possess anti-inflammatory, antioxidant, and antimicrobial activities, among others. In recent years, it has also been investigated for its potential role in the treatment of various diseases and disorders, such as cancer, diabetes, and neurodegenerative diseases.
科学研究应用
Drug Toxicity Mediation
Acyl glucuronides, including Acyl SA glucuronide, have been implicated in the mediation of drug-induced toxicities. They are reactive drug conjugates that can covalently modify biological molecules such as proteins, lipids, and nucleic acids . This reactivity is due to their ability to undergo transacylation reactions with nucleophilic centers on macromolecules or glycation of protein residues . Understanding the role of Acyl SA glucuronide in these processes is crucial for assessing the safety of drugs that metabolize into this compound.
Inhibition of Key Enzymes and Transporters
Studies have shown that Acyl SA glucuronide can inhibit key enzymes and transporters, which may lead to the accumulation of the conjugate in liver tissue . This inhibition is significant because it can affect the pharmacokinetics of drugs and potentially lead to adverse drug reactions. Research into the specific enzymes and transporters affected by Acyl SA glucuronide is ongoing to better understand its impact on drug metabolism and toxicity.
Kinetic Modelling and Reactivity Prediction
Kinetic modelling of Acyl SA glucuronide’s reactivity has been developed to predict its behavior in biological systems . This includes understanding its degradation via intramolecular transacylation and hydrolysis, which is associated with the formation of protein adducts . Such models are valuable in drug discovery for predicting the potential hepatotoxicity of new drugs.
Analytical Methodology Advancements
The inherent instability of Acyl SA glucuronide under physiological conditions has led to advancements in analytical methodologies used to detect, identify, and quantify this metabolite in biological fluids and tissues . These methodologies are essential for studying the pharmacokinetics and dynamics of drugs that form Acyl SA glucuronide as a metabolite.
De-risking Strategies in Drug Discovery
Understanding the molecular mechanisms of Acyl SA glucuronide’s toxicity has led to the adoption of de-risking strategies in drug discovery and lead optimization programs . These strategies aim to minimize the likelihood of Acyl SA glucuronide-mediated toxicity by identifying and avoiding the development of drugs that are likely to form highly reactive glucuronide metabolites.
Structural Analysis and Chemical Shift Calculation
Research has also focused on the molecular structure of Acyl SA glucuronide for calculating NMR chemical shifts and other electronic parameters . This structural analysis is important for understanding the electronic environment of the compound, which influences its reactivity and interactions with biological molecules.
作用机制
Target of Action
Acyl SA glucuronide, also known as Salicyl acyl glucuronide, is a metabolite of carboxylic acid-containing drugs . Its primary targets are biological molecules, including proteins, lipids, and nucleic acids . It has the ability to covalently modify these targets through transacylation of nucleophilic centers on macromolecules . Additionally, it can inhibit key enzymes and transporters, such as OATP1B1/3 (hepatic) and OAT1/3 (renal), and efflux transporters like MRP2, BSEP, and BCRP on the bile canalicular membrane, and MRP3/4 on the basolateral membrane of hepatocytes .
Mode of Action
The mode of action of Acyl SA glucuronide involves its interaction with its targets, leading to significant changes. It exhibits a degree of electrophilicity, strongly influenced by the attached drug moiety in terms of the steric and electronic environment of the ester linkage to the sugar . This allows it to covalently modify biological molecules . These modifications occur either by way of transacylation of nucleophilic centers on macromolecules, such as the -OH, -NH2, and -SH groups of proteins, or by glycation of protein -NH2 residues through spontaneous intramolecular migration of the drug-acyl moiety of the conjugate .
Biochemical Pathways
The formation of Acyl SA glucuronide is a result of the conjugation of glucuronic acid, an important pathway in the metabolism of carboxylic acid-containing drugs in both animals and humans . This results in the formation of 1-β-O-acyl-glucuronide ester derivatives that often circulate in plasma prior to being excreted in urine and bile . The biochemical pathways affected by Acyl SA glucuronide are those involving the targets it modifies, including various enzymatic and transporter pathways .
Pharmacokinetics
The pharmacokinetics of Acyl SA glucuronide involve its absorption, distribution, metabolism, and excretion (ADME). As a metabolite, it is formed in the body through the metabolism of carboxylic acid-containing drugs . It circulates in plasma before being excreted in urine and bile . The inherent instability of Acyl SA glucuronide under physiological conditions can lead to significant challenges in assessing its bioavailability .
Result of Action
The result of Acyl SA glucuronide’s action is the modification of biological molecules, including proteins, lipids, and nucleic acids . This can lead to changes in the function of these molecules and potentially contribute to drug-induced toxicities . The degree of protein adduct formation has been associated with the rate of degradation of Acyl SA glucuronide via intramolecular transacylation and hydrolysis .
Action Environment
The action of Acyl SA glucuronide can be influenced by various environmental factors. Its inherent instability under physiological conditions can affect its action, efficacy, and stability . Furthermore, the structure of the particular Acyl SA glucuronide can influence which transporters are affected . Understanding these factors is crucial for assessing the safety and efficacy of carboxylic acid-containing drugs and their Acyl SA glucuronide metabolites .
属性
IUPAC Name |
(2S,3S,4S,5R,6S)-3,4,5-trihydroxy-6-(2-hydroxybenzoyl)oxyoxane-2-carboxylic acid | |
---|---|---|
Source | PubChem | |
URL | https://pubchem.ncbi.nlm.nih.gov | |
Description | Data deposited in or computed by PubChem | |
InChI |
InChI=1S/C13H14O9/c14-6-4-2-1-3-5(6)12(20)22-13-9(17)7(15)8(16)10(21-13)11(18)19/h1-4,7-10,13-17H,(H,18,19)/t7-,8-,9+,10-,13-/m0/s1 | |
Source | PubChem | |
URL | https://pubchem.ncbi.nlm.nih.gov | |
Description | Data deposited in or computed by PubChem | |
InChI Key |
IXVVXKRKCLJCKA-UNLLLRGISA-N | |
Source | PubChem | |
URL | https://pubchem.ncbi.nlm.nih.gov | |
Description | Data deposited in or computed by PubChem | |
Canonical SMILES |
C1=CC=C(C(=C1)C(=O)OC2C(C(C(C(O2)C(=O)O)O)O)O)O | |
Source | PubChem | |
URL | https://pubchem.ncbi.nlm.nih.gov | |
Description | Data deposited in or computed by PubChem | |
Isomeric SMILES |
C1=CC=C(C(=C1)C(=O)O[C@H]2[C@@H]([C@H]([C@@H]([C@H](O2)C(=O)O)O)O)O)O | |
Source | PubChem | |
URL | https://pubchem.ncbi.nlm.nih.gov | |
Description | Data deposited in or computed by PubChem | |
Molecular Formula |
C13H14O9 | |
Source | PubChem | |
URL | https://pubchem.ncbi.nlm.nih.gov | |
Description | Data deposited in or computed by PubChem | |
DSSTOX Substance ID |
DTXSID70951878 | |
Record name | 1-O-(2-Hydroxybenzoyl)hexopyranuronic acid | |
Source | EPA DSSTox | |
URL | https://comptox.epa.gov/dashboard/DTXSID70951878 | |
Description | DSSTox provides a high quality public chemistry resource for supporting improved predictive toxicology. | |
Molecular Weight |
314.24 g/mol | |
Source | PubChem | |
URL | https://pubchem.ncbi.nlm.nih.gov | |
Description | Data deposited in or computed by PubChem | |
Physical Description |
Solid | |
Record name | Beta-D-Glucopyranuronic acid | |
Source | Human Metabolome Database (HMDB) | |
URL | http://www.hmdb.ca/metabolites/HMDB0010314 | |
Description | The Human Metabolome Database (HMDB) is a freely available electronic database containing detailed information about small molecule metabolites found in the human body. | |
Explanation | HMDB is offered to the public as a freely available resource. Use and re-distribution of the data, in whole or in part, for commercial purposes requires explicit permission of the authors and explicit acknowledgment of the source material (HMDB) and the original publication (see the HMDB citing page). We ask that users who download significant portions of the database cite the HMDB paper in any resulting publications. | |
Product Name |
(2S,3S,4S,5R,6S)-3,4,5-trihydroxy-6-(2-hydroxybenzoyl)oxyoxane-2-carboxylic acid | |
CAS RN |
29315-53-5 | |
Record name | Salicyl acyl glucuronide | |
Source | CAS Common Chemistry | |
URL | https://commonchemistry.cas.org/detail?cas_rn=29315-53-5 | |
Description | CAS Common Chemistry is an open community resource for accessing chemical information. Nearly 500,000 chemical substances from CAS REGISTRY cover areas of community interest, including common and frequently regulated chemicals, and those relevant to high school and undergraduate chemistry classes. This chemical information, curated by our expert scientists, is provided in alignment with our mission as a division of the American Chemical Society. | |
Explanation | The data from CAS Common Chemistry is provided under a CC-BY-NC 4.0 license, unless otherwise stated. | |
Record name | Salicylacyl glucuronide | |
Source | ChemIDplus | |
URL | https://pubchem.ncbi.nlm.nih.gov/substance/?source=chemidplus&sourceid=0029315535 | |
Description | ChemIDplus is a free, web search system that provides access to the structure and nomenclature authority files used for the identification of chemical substances cited in National Library of Medicine (NLM) databases, including the TOXNET system. | |
Record name | 1-O-(2-Hydroxybenzoyl)hexopyranuronic acid | |
Source | EPA DSSTox | |
URL | https://comptox.epa.gov/dashboard/DTXSID70951878 | |
Description | DSSTox provides a high quality public chemistry resource for supporting improved predictive toxicology. | |
Record name | Beta-D-Glucopyranuronic acid | |
Source | Human Metabolome Database (HMDB) | |
URL | http://www.hmdb.ca/metabolites/HMDB0010314 | |
Description | The Human Metabolome Database (HMDB) is a freely available electronic database containing detailed information about small molecule metabolites found in the human body. | |
Explanation | HMDB is offered to the public as a freely available resource. Use and re-distribution of the data, in whole or in part, for commercial purposes requires explicit permission of the authors and explicit acknowledgment of the source material (HMDB) and the original publication (see the HMDB citing page). We ask that users who download significant portions of the database cite the HMDB paper in any resulting publications. | |
Retrosynthesis Analysis
AI-Powered Synthesis Planning: Our tool employs the Template_relevance Pistachio, Template_relevance Bkms_metabolic, Template_relevance Pistachio_ringbreaker, Template_relevance Reaxys, Template_relevance Reaxys_biocatalysis model, leveraging a vast database of chemical reactions to predict feasible synthetic routes.
One-Step Synthesis Focus: Specifically designed for one-step synthesis, it provides concise and direct routes for your target compounds, streamlining the synthesis process.
Accurate Predictions: Utilizing the extensive PISTACHIO, BKMS_METABOLIC, PISTACHIO_RINGBREAKER, REAXYS, REAXYS_BIOCATALYSIS database, our tool offers high-accuracy predictions, reflecting the latest in chemical research and data.
Strategy Settings
Precursor scoring | Relevance Heuristic |
---|---|
Min. plausibility | 0.01 |
Model | Template_relevance |
Template Set | Pistachio/Bkms_metabolic/Pistachio_ringbreaker/Reaxys/Reaxys_biocatalysis |
Top-N result to add to graph | 6 |
Feasible Synthetic Routes
体外研究产品的免责声明和信息
请注意,BenchChem 上展示的所有文章和产品信息仅供信息参考。 BenchChem 上可购买的产品专为体外研究设计,这些研究在生物体外进行。体外研究,源自拉丁语 "in glass",涉及在受控实验室环境中使用细胞或组织进行的实验。重要的是要注意,这些产品没有被归类为药物或药品,他们没有得到 FDA 的批准,用于预防、治疗或治愈任何医疗状况、疾病或疾病。我们必须强调,将这些产品以任何形式引入人类或动物的身体都是法律严格禁止的。遵守这些指南对确保研究和实验的法律和道德标准的符合性至关重要。